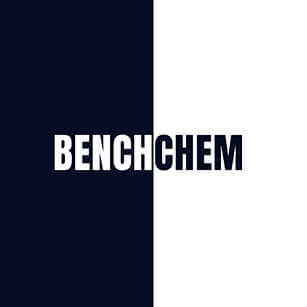
LY249543 disodium
- 点击 快速询问 获取最新报价。
- 提供有竞争力价格的高质量产品,您可以更专注于研究。
描述
准备方法
The synthesis of LY249543 disodium involves the reaction of specific precursors under controlled conditions. The detailed synthetic routes and reaction conditions are proprietary and not widely disclosed in public literature. it is known that the compound is synthesized in a laboratory setting and requires precise control of temperature, pH, and other reaction parameters . Industrial production methods are also not publicly available, but they likely involve scaling up the laboratory synthesis process while ensuring consistency and purity of the final product .
化学反应分析
LY249543 disodium undergoes various chemical reactions, including:
Oxidation: This reaction involves the addition of oxygen or the removal of hydrogen. Common oxidizing agents include potassium permanganate and hydrogen peroxide.
Reduction: This reaction involves the addition of hydrogen or the removal of oxygen. Common reducing agents include sodium borohydride and lithium aluminum hydride.
Substitution: This reaction involves the replacement of one atom or group of atoms with another.
The major products formed from these reactions depend on the specific conditions and reagents used. For example, oxidation may result in the formation of carboxylic acids, while reduction may yield alcohols .
科学研究应用
LY249543 disodium has several scientific research applications, including:
Chemistry: It is used as a research tool to study the inhibition of folate metabolism and its effects on cellular processes.
Biology: It is used to investigate the role of folate metabolism in cell growth and proliferation.
作用机制
LY249543 disodium exerts its effects by inhibiting Methylenetetrahydrofolate Dehydrogenase/Cyclohydrolase, an enzyme involved in folate metabolism. This inhibition disrupts the synthesis of tetrahydrofolate, a cofactor required for the synthesis of nucleotides and amino acids. As a result, the compound interferes with DNA synthesis and cell division, leading to the inhibition of cell growth and proliferation .
相似化合物的比较
LY249543 disodium is similar to other antifolate compounds, such as methotrexate and pemetrexed. it is unique in its specific inhibition of Methylenetetrahydrofolate Dehydrogenase/Cyclohydrolase. Other similar compounds include:
Methotrexate: Inhibits dihydrofolate reductase.
Pemetrexed: Inhibits multiple enzymes involved in folate metabolism, including thymidylate synthase and dihydrofolate reductase.
This compound’s specificity for Methylenetetrahydrofolate Dehydrogenase/Cyclohydrolase makes it a valuable tool for studying the role of this enzyme in cellular processes and for developing targeted therapies .
属性
CAS 编号 |
106400-18-4 |
---|---|
分子式 |
C21H23N5Na2O6 |
分子量 |
487.4235 |
IUPAC 名称 |
disodium (4-(2-((S)-2-amino-4-oxo-1,4,5,6,7,8-hexahydropyrido[2,3-d]pyrimidin-6-yl)ethyl)benzoyl)-L-glutamate |
InChI |
InChI=1S/C21H25N5O6.2Na/c22-21-25-17-14(19(30)26-21)9-12(10-23-17)2-1-11-3-5-13(6-4-11)18(29)24-15(20(31)32)7-8-16(27)28;;/h3-6,12,15H,1-2,7-10H2,(H,24,29)(H,27,28)(H,31,32)(H4,22,23,25,26,30);;/q;2*+1/p-2/t12-,15-;;/m0../s1 |
InChI 键 |
SVJSWELRJWVPQD-XPTKPXFUSA-L |
SMILES |
O=C([O-])CC[C@@H](C([O-])=O)NC(C1=CC=C(CC[C@H](CN2)CC3=C2NC(N)=NC3=O)C=C1)=O.[Na+].[Na+] |
外观 |
Solid powder |
纯度 |
>98% (or refer to the Certificate of Analysis) |
保质期 |
>2 years if stored properly |
溶解度 |
Soluble in DMSO |
储存 |
Dry, dark and at 0 - 4 C for short term (days to weeks) or -20 C for long term (months to years). |
同义词 |
LY249543; LY 249543; LY-249543; LY249543 sodium; S-isomer of lometrexol; DB04322; lometrexol S-isomer. |
产品来源 |
United States |
Retrosynthesis Analysis
AI-Powered Synthesis Planning: Our tool employs the Template_relevance Pistachio, Template_relevance Bkms_metabolic, Template_relevance Pistachio_ringbreaker, Template_relevance Reaxys, Template_relevance Reaxys_biocatalysis model, leveraging a vast database of chemical reactions to predict feasible synthetic routes.
One-Step Synthesis Focus: Specifically designed for one-step synthesis, it provides concise and direct routes for your target compounds, streamlining the synthesis process.
Accurate Predictions: Utilizing the extensive PISTACHIO, BKMS_METABOLIC, PISTACHIO_RINGBREAKER, REAXYS, REAXYS_BIOCATALYSIS database, our tool offers high-accuracy predictions, reflecting the latest in chemical research and data.
Strategy Settings
Precursor scoring | Relevance Heuristic |
---|---|
Min. plausibility | 0.01 |
Model | Template_relevance |
Template Set | Pistachio/Bkms_metabolic/Pistachio_ringbreaker/Reaxys/Reaxys_biocatalysis |
Top-N result to add to graph | 6 |
Feasible Synthetic Routes
体外研究产品的免责声明和信息
请注意,BenchChem 上展示的所有文章和产品信息仅供信息参考。 BenchChem 上可购买的产品专为体外研究设计,这些研究在生物体外进行。体外研究,源自拉丁语 "in glass",涉及在受控实验室环境中使用细胞或组织进行的实验。重要的是要注意,这些产品没有被归类为药物或药品,他们没有得到 FDA 的批准,用于预防、治疗或治愈任何医疗状况、疾病或疾病。我们必须强调,将这些产品以任何形式引入人类或动物的身体都是法律严格禁止的。遵守这些指南对确保研究和实验的法律和道德标准的符合性至关重要。