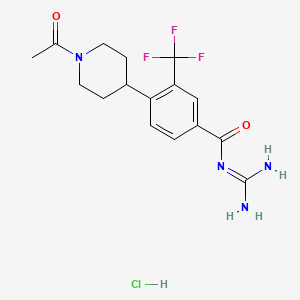
BIX NHE1 抑制剂
描述
科学研究应用
BIX Hydrochloride has a wide range of applications in scientific research:
Chemistry: Used as a reagent in various chemical reactions to study the inhibition of sodium-hydrogen exchange.
Biology: Employed in cell biology to investigate the role of NHE1 in regulating intracellular pH and cell volume.
Medicine: Explored for its potential therapeutic applications in conditions where NHE1 activity is dysregulated, such as in certain cardiovascular and cancer treatments.
Industry: Utilized in the development of new drugs and therapeutic agents targeting NHE1.
作用机制
- NHE1 is a transmembrane ion channel expressed ubiquitously in cells. Its main function is to regulate intracellular pH by exchanging sodium ions (Na⁺) and protons (H⁺) in an electroneutral manner .
- BIX NHE1 inhibitor selectively inhibits NHE1 with an IC₅₀ of 31 nM .
- This inhibition affects cell volume regulation and water influx, which plays a role in cell migration .
Target of Action
Mode of Action
Pharmacokinetics
Result of Action
生化分析
Biochemical Properties
BIX NHE1 inhibitor interacts with the NHE1 isoform, a transmembrane ion channel present on the plasma membrane of most mammalian cells . It inhibits NHE1 with IC50 values of 6 and 31 nM in intracellular pH recovery and human platelet swelling assays, respectively . It displays over 30-fold selectivity against NHE2 and shows no measurable inhibitory activity against the NHE3 isoform .
Cellular Effects
The BIX NHE1 inhibitor has significant effects on various types of cells and cellular processes. It prevents ischemic damage in an ischemia reperfusion injury isolated rat heart model ex vivo . It also prevents phenylephrine-induced cardiomyocyte hypertrophy in vitro, and attenuates cardiac hypertrophy and left ventricular dysfunction postinfarction in rats . In neutrophils, cell swelling induced by the BIX NHE1 inhibitor is both necessary and sufficient for the potentiation of migration following chemoattractant stimulation .
Molecular Mechanism
The BIX NHE1 inhibitor exerts its effects at the molecular level through various mechanisms. It binds to the NHE1 isoform, inhibiting its function and leading to changes in intracellular pH and volume . This binding interaction is crucial for its inhibitory activity.
Temporal Effects in Laboratory Settings
In laboratory settings, the effects of the BIX NHE1 inhibitor change over time. It shows low DDI (agent-agent interaction) potential, excellent pharmacokinetics in rat and dog, and remarkably potent activity in the isolated heart model of ischemia-reperfusion injury . Information on the product’s stability, degradation, and any long-term effects on cellular function observed in in vitro or in vivo studies is currently limited.
Metabolic Pathways
The BIX NHE1 inhibitor is involved in the regulation of hydrogen ion dynamics, a critical metabolic pathway in cells . It interacts with the NHE1 isoform, a key enzyme in this pathway
Transport and Distribution
The BIX NHE1 inhibitor is transported and distributed within cells and tissues, likely through its interaction with the NHE1 isoform located on the plasma membrane
Subcellular Localization
The BIX NHE1 inhibitor primarily interacts with the NHE1 isoform, which is located on the plasma membrane of most mammalian cells
准备方法
Synthetic Routes and Reaction Conditions
The synthesis of BIX Hydrochloride involves multiple steps, starting from the appropriate precursor compounds. The detailed synthetic route and reaction conditions are typically proprietary information held by the manufacturers. general laboratory preparation involves dissolving the compound in solvents like dimethyl sulfoxide (DMSO) and polyethylene glycol (PEG300), followed by the addition of surfactants like Tween 80 and distilled water to achieve the desired concentration .
Industrial Production Methods
Industrial production methods for BIX Hydrochloride are not widely published, but they likely involve large-scale synthesis using similar principles as laboratory preparation, with additional steps for purification and quality control to ensure consistency and potency.
化学反应分析
Types of Reactions
BIX Hydrochloride primarily undergoes substitution reactions due to its functional groups. It can also participate in acid-base reactions, given its hydrochloride form.
Common Reagents and Conditions
Common reagents used in reactions involving BIX Hydrochloride include strong acids and bases, organic solvents like DMSO, and various surfactants for solubilization. The reaction conditions typically involve controlled temperatures and pH to maintain the stability of the compound .
Major Products Formed
The major products formed from reactions involving BIX Hydrochloride depend on the specific reagents and conditions used
相似化合物的比较
Similar Compounds
Ambroxol Hydrochloride: Used as a mucolytic agent in respiratory conditions.
Bromhexine Hydrochloride: Another mucolytic agent with similar applications to Ambroxol Hydrochloride.
Uniqueness
BIX Hydrochloride is unique in its specific inhibition of the sodium-hydrogen exchanger 1 (NHE1), which sets it apart from other hydrochloride compounds that may have different targets and applications. Its role in regulating intracellular pH and cell volume makes it particularly valuable in research focused on cellular homeostasis and related therapeutic applications .
属性
IUPAC Name |
4-(1-acetylpiperidin-4-yl)-N-(diaminomethylidene)-3-(trifluoromethyl)benzamide;hydrochloride | |
---|---|---|
Source | PubChem | |
URL | https://pubchem.ncbi.nlm.nih.gov | |
Description | Data deposited in or computed by PubChem | |
InChI |
InChI=1S/C16H19F3N4O2.ClH/c1-9(24)23-6-4-10(5-7-23)12-3-2-11(14(25)22-15(20)21)8-13(12)16(17,18)19;/h2-3,8,10H,4-7H2,1H3,(H4,20,21,22,25);1H | |
Source | PubChem | |
URL | https://pubchem.ncbi.nlm.nih.gov | |
Description | Data deposited in or computed by PubChem | |
InChI Key |
SLSZLEROFKLICF-UHFFFAOYSA-N | |
Source | PubChem | |
URL | https://pubchem.ncbi.nlm.nih.gov | |
Description | Data deposited in or computed by PubChem | |
Canonical SMILES |
CC(=O)N1CCC(CC1)C2=C(C=C(C=C2)C(=O)N=C(N)N)C(F)(F)F.Cl | |
Source | PubChem | |
URL | https://pubchem.ncbi.nlm.nih.gov | |
Description | Data deposited in or computed by PubChem | |
Molecular Formula |
C16H20ClF3N4O2 | |
Source | PubChem | |
URL | https://pubchem.ncbi.nlm.nih.gov | |
Description | Data deposited in or computed by PubChem | |
Molecular Weight |
392.80 g/mol | |
Source | PubChem | |
URL | https://pubchem.ncbi.nlm.nih.gov | |
Description | Data deposited in or computed by PubChem | |
Retrosynthesis Analysis
AI-Powered Synthesis Planning: Our tool employs the Template_relevance Pistachio, Template_relevance Bkms_metabolic, Template_relevance Pistachio_ringbreaker, Template_relevance Reaxys, Template_relevance Reaxys_biocatalysis model, leveraging a vast database of chemical reactions to predict feasible synthetic routes.
One-Step Synthesis Focus: Specifically designed for one-step synthesis, it provides concise and direct routes for your target compounds, streamlining the synthesis process.
Accurate Predictions: Utilizing the extensive PISTACHIO, BKMS_METABOLIC, PISTACHIO_RINGBREAKER, REAXYS, REAXYS_BIOCATALYSIS database, our tool offers high-accuracy predictions, reflecting the latest in chemical research and data.
Strategy Settings
Precursor scoring | Relevance Heuristic |
---|---|
Min. plausibility | 0.01 |
Model | Template_relevance |
Template Set | Pistachio/Bkms_metabolic/Pistachio_ringbreaker/Reaxys/Reaxys_biocatalysis |
Top-N result to add to graph | 6 |
Feasible Synthetic Routes
体外研究产品的免责声明和信息
请注意,BenchChem 上展示的所有文章和产品信息仅供信息参考。 BenchChem 上可购买的产品专为体外研究设计,这些研究在生物体外进行。体外研究,源自拉丁语 "in glass",涉及在受控实验室环境中使用细胞或组织进行的实验。重要的是要注意,这些产品没有被归类为药物或药品,他们没有得到 FDA 的批准,用于预防、治疗或治愈任何医疗状况、疾病或疾病。我们必须强调,将这些产品以任何形式引入人类或动物的身体都是法律严格禁止的。遵守这些指南对确保研究和实验的法律和道德标准的符合性至关重要。