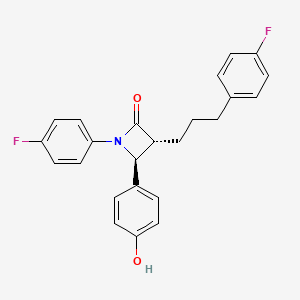
Ezetimibe Dehydoxy Impurity
描述
Ezetimibe Dehydoxy Impurity is a process-related impurity identified during the synthesis of ezetimibe, a cholesterol absorption inhibitor used to treat hyperlipidemia. This impurity arises due to the loss of a hydroxyl group (-OH) from the parent molecule, altering its stereochemical and physicochemical properties. Structurally, it is defined as 3-Dehydroxy Ezetimibe (C₂₄H₂₁F₂NO₂; molecular weight: 393.44 g/mol) . Its presence in drug substances is tightly regulated, as per ICH guidelines, which mandate identification and characterization of impurities exceeding 0.10% .
The impurity is synthesized via pathways analogous to ezetimibe but involves deviations in hydroxylation steps during intermediate formation. Analytical techniques such as HPLC, NMR (¹H, ¹³C, DEPT), and mass spectrometry confirm its identity and purity . Control of this impurity is critical, as it cannot be removed through recrystallization and must be minimized during early synthetic stages .
准备方法
The preparation of Ezetimibe Dehydoxy Impurity involves synthetic routes and reaction conditions similar to those used for Ezetimibe. One common method involves the degradation of Ezetimibe under alkaline conditions. For instance, Ezetimibe can be heated with 0.01 M aqueous sodium hydroxide solution at 60°C for 10 minutes, leading to the formation of degradation products, including this compound . Industrial production methods for such impurities typically involve controlled degradation processes and isolation using techniques like high-performance liquid chromatography (HPLC).
化学反应分析
Ezetimibe Dehydoxy Impurity undergoes various chemical reactions, including:
Oxidation: This reaction involves the addition of oxygen or the removal of hydrogen. Common reagents include hydrogen peroxide and potassium permanganate.
Reduction: This reaction involves the addition of hydrogen or the removal of oxygen. Common reagents include sodium borohydride and lithium aluminum hydride.
Substitution: This reaction involves the replacement of one atom or group of atoms with another. Common reagents include halogens and nucleophiles.
The major products formed from these reactions depend on the specific conditions and reagents used. For example, oxidation of this compound can lead to the formation of various oxidized derivatives .
科学研究应用
Synthesis and Characterization
The synthesis of Ezetimibe Dehydoxy Impurity typically involves degradation reactions under specific conditions. Research indicates that this impurity can be generated through alkaline hydrolysis or oxidation processes. For instance, one method involves dissolving Ezetimibe in a solvent and adding an alkali, followed by controlled reaction conditions to yield high-purity degradation products .
Analytical Techniques
Characterization of this compound is performed using various analytical techniques:
- High-Performance Liquid Chromatography (HPLC) : Used for quantifying the impurity levels in drug formulations.
- Mass Spectrometry (MS) : Helps confirm the molecular structure of the impurity.
- Nuclear Magnetic Resonance (NMR) Spectroscopy : Provides detailed structural information about the impurity.
Regulatory Compliance and Quality Control
The presence of impurities like this compound must be monitored to comply with regulatory guidelines set by organizations such as the International Conference on Harmonization (ICH). These guidelines mandate that all identified and unidentified impurities be reported, characterized, and their levels controlled to ensure product safety and efficacy .
Impurity Profiling
Impurity profiling involves systematic studies to identify both known and unknown impurities in drug substances. This process is crucial in the development phase to establish acceptable limits for impurities, thereby ensuring that they do not exceed regulatory thresholds during production .
Case Studies
Several case studies highlight the importance of monitoring this compound:
- Case Study 1 : A study demonstrated that the alkaline degradant of Ezetimibe was isolated with a purity exceeding 99%. The structure was confirmed using LC-MS and NMR techniques, showcasing how effective impurity profiling can enhance drug safety .
- Case Study 2 : Another investigation focused on the synthesis of various process-related impurities, including this compound. The study emphasized the need for rigorous analytical methods to ensure that impurities remain within acceptable limits throughout the drug's shelf life .
Applications in Drug Development
This compound has several applications in pharmaceutical research:
- Quality Assurance : Regular monitoring helps maintain high-quality standards for Ezetimibe formulations.
- Stability Testing : Understanding how this impurity forms under different conditions aids in developing stable formulations.
- Therapeutic Insights : Research into impurities can provide insights into the pharmacological effects of Ezetimibe, potentially leading to improved therapeutic strategies for managing hyperlipidemia.
作用机制
相似化合物的比较
Structural and Functional Differences
The table below compares Ezetimibe Dehydoxy Impurity with other key impurities associated with ezetimibe synthesis:
Key Observations:
Desfluoro Ezetimibe is structurally closest to the Dehydoxy Impurity but differs in halogen substitution. It forms due to fluorine loss in intermediate Eze-1 and is controlled at a higher threshold (≤0.15%) .
Aniline Impurity originates from unreacted starting materials, requiring stringent purification to meet ICH limits .
Azetidinone Ring-Opened Impurity is a degradation product, necessitating stability-indicating methods for detection .
Analytical Method Performance
- HPLC Resolution :
- Recovery and Precision :
Research Findings and Regulatory Implications
Degradation Resistance: Unlike degradation-related impurities (e.g., azetidinone ring-opened), Dehydoxy and Desfluoro impurities are process-related and persist through synthesis, necessitating in-process controls .
Method Robustness : Multi-technique validation (NMR, IR, MS) ensures unambiguous identification, critical for regulatory filings .
Commercial Standards : Suppliers such as SynZeal and SynThink offer Dehydoxy Impurity with ≥97.9% purity, enabling compliance with pharmacopeial requirements .
生物活性
Ezetimibe Dehydoxy Impurity, a degradation product of the cholesterol absorption inhibitor ezetimibe, has gained attention in pharmaceutical research due to its potential biological activities and implications in drug formulation. This article delves into the biological activity of this compound, exploring its mechanisms, biochemical interactions, and relevant case studies.
This compound is characterized by its molecular structure, which includes a fluorophenyl group and a hydroxyphenyl moiety. The compound is typically formed through the removal of a hydroxyl group from ezetimibe during degradation processes .
Table 1: Chemical Properties of this compound
Property | Value |
---|---|
Molecular Formula | C₁₈H₁₈F₁N₁O₂ |
Molecular Weight | 295.34 g/mol |
Melting Point | Not specified |
Boiling Point | Not specified |
Solubility | Soluble in organic solvents |
Inhibition of Cholesterol Absorption
Research indicates that this compound may exhibit similar mechanisms to its parent compound in inhibiting cholesterol absorption. This activity can be assessed through various biochemical assays that measure changes in cholesterol levels in cell cultures or animal models.
In a study involving hamsters, ezetimibe demonstrated significant reductions in low-density lipoprotein (LDL) cholesterol levels while enhancing reverse cholesterol transport . Although specific data on this compound is sparse, it is reasonable to infer potential atheroprotective effects based on its structural similarity to ezetimibe.
Case Studies
- Cholesterol-Fed Hamsters : In experiments where hamsters were fed diets supplemented with cholesterol and treated with ezetimibe, significant reductions in plasma total cholesterol were observed. Dosages as low as 0.03 mg/kg/day showed efficacy in lowering LDL levels .
- Human Clinical Trials : While direct clinical data on this compound is lacking, studies on ezetimibe have shown improvements in surrogate markers for cardiovascular health, including reductions in LDL-C and improvements in glucose metabolism . These findings suggest that related impurities might also contribute positively to metabolic profiles.
Biochemical Interactions
Ezetimibe and its impurities likely interact with various enzymes involved in lipid metabolism. The inhibition of NPC1L1 leads to decreased intestinal absorption of cholesterol, which may subsequently affect hepatic lipid profiles and overall lipid homeostasis.
Table 2: Comparative Biological Activities
Compound | Mechanism of Action | Biological Effects |
---|---|---|
Ezetimibe | NPC1L1 inhibition | Reduced LDL-C, enhanced reverse transport |
This compound | Hypothetical NPC1L1 inhibition | Potentially similar effects as ezetimibe |
常见问题
Basic Research Questions
Q. What analytical methods are recommended for identifying and quantifying Ezetimibe Dehydoxy Impurity in drug substances?
A validated High-Performance Liquid Chromatography (HPLC) method is commonly employed for quantification, as it effectively separates known and unknown impurities in ezetimibe formulations . For structural confirmation, techniques such as Nuclear Magnetic Resonance (NMR) and Mass Spectrometry (LC-MS/MS) are critical. For example, NMR (¹H and ¹³C) and ESI-MS were used to verify the structure of synthesized impurities, including this compound, with HPLC ensuring purity ≥98% . Method validation should adhere to pharmacopeial guidelines, including specificity, linearity, and precision testing .
Q. How is this compound synthesized for use as a reference standard?
The impurity can be synthesized via selective oxidation or hydrogenation of ezetimibe intermediates. For instance, pyridinium chlorochromate (PCC) oxidation of ezetimibe yields related ketone impurities, while catalytic hydrogenation of intermediates produces structural analogs like the Dehydoxy variant . Strict control of reaction conditions (e.g., temperature, catalyst loading) is essential to minimize side products.
Q. What regulatory thresholds govern the acceptance of this compound in drug products?
Per the United States Pharmacopoeia (USP), individual unspecified impurities in ezetimibe formulations must not exceed 0.20%, with total impurities capped at 0.60% . Compliance requires rigorous batch testing using validated methods to ensure impurity profiles align with these limits.
Q. How does batch-to-batch variability in ezetimibe synthesis impact Dehydoxy Impurity levels?
Variability arises from differences in starting materials, reaction conditions, and purification steps. For example, batches using divergent ezetimibe active ingredient sources may exhibit qualitative and quantitative impurity differences . Statistical process control (SPC) and Design of Experiments (DoE) can identify critical process parameters affecting impurity formation .
Advanced Research Questions
Q. How can researchers design experiments to investigate factors influencing this compound formation?
A factorial design approach is recommended to evaluate interactions between variables such as temperature, pH, and catalyst concentration. For example, a 2³ factorial design could test these factors at high/low levels to identify significant contributors to impurity formation . Data analysis using ANOVA or multivariate regression models can isolate critical parameters .
Q. What strategies resolve contradictions in impurity profiles between synthetic and degradation studies?
Discrepancies often stem from divergent formation pathways (synthesis vs. storage degradation). Mechanistic studies using forced degradation (e.g., heat, light, humidity) coupled with LC-MS/MS can differentiate process-related impurities (e.g., Dehydoxy Impurity) from degradants . Cross-referencing synthetic impurity data with stability studies clarifies origins .
Q. How can genotoxic risk assessments be integrated into impurity analysis for this compound?
While this compound is not explicitly classified as genotoxic, its structural analogs (e.g., aniline derivatives) may pose risks. Employ in silico tools (e.g., QSAR models) for initial screening, followed by in vitro assays (Ames test) if alerts are detected . Analytical methods must achieve detection limits ≤1 ppm for genotoxic impurities .
Q. What mechanistic insights explain the low prevalence of ring-opened impurities in ezetimibe formulations?
Ezetimibe’s β-lactam analogs are resistant to ring-opening under standard manufacturing conditions. Impurity D (a synthesis intermediate) is the sole ring-opened impurity documented, controlled via stringent API specifications . Kinetic studies using Arrhenius modeling can predict degradation pathways under stress conditions .
Q. Methodological Recommendations
- For Batch Comparisons: Use principal component analysis (PCA) to visualize impurity profile variability across batches .
- For Synthetic Optimization: Apply Quality by Design (QbD) principles to define the design space for impurity control .
- For Data Integrity: Incorporate open-ended questions and attention checks in experimental logs to mitigate analytical bias .
属性
IUPAC Name |
(3R,4S)-1-(4-fluorophenyl)-3-[3-(4-fluorophenyl)propyl]-4-(4-hydroxyphenyl)azetidin-2-one | |
---|---|---|
Source | PubChem | |
URL | https://pubchem.ncbi.nlm.nih.gov | |
Description | Data deposited in or computed by PubChem | |
InChI |
InChI=1S/C24H21F2NO2/c25-18-8-4-16(5-9-18)2-1-3-22-23(17-6-14-21(28)15-7-17)27(24(22)29)20-12-10-19(26)11-13-20/h4-15,22-23,28H,1-3H2/t22-,23-/m1/s1 | |
Source | PubChem | |
URL | https://pubchem.ncbi.nlm.nih.gov | |
Description | Data deposited in or computed by PubChem | |
InChI Key |
GPGSMLXOBVGHQH-DHIUTWEWSA-N | |
Source | PubChem | |
URL | https://pubchem.ncbi.nlm.nih.gov | |
Description | Data deposited in or computed by PubChem | |
Canonical SMILES |
C1=CC(=CC=C1CCCC2C(N(C2=O)C3=CC=C(C=C3)F)C4=CC=C(C=C4)O)F | |
Source | PubChem | |
URL | https://pubchem.ncbi.nlm.nih.gov | |
Description | Data deposited in or computed by PubChem | |
Isomeric SMILES |
C1=CC(=CC=C1CCC[C@@H]2[C@H](N(C2=O)C3=CC=C(C=C3)F)C4=CC=C(C=C4)O)F | |
Source | PubChem | |
URL | https://pubchem.ncbi.nlm.nih.gov | |
Description | Data deposited in or computed by PubChem | |
Molecular Formula |
C24H21F2NO2 | |
Source | PubChem | |
URL | https://pubchem.ncbi.nlm.nih.gov | |
Description | Data deposited in or computed by PubChem | |
Molecular Weight |
393.4 g/mol | |
Source | PubChem | |
URL | https://pubchem.ncbi.nlm.nih.gov | |
Description | Data deposited in or computed by PubChem | |
Retrosynthesis Analysis
AI-Powered Synthesis Planning: Our tool employs the Template_relevance Pistachio, Template_relevance Bkms_metabolic, Template_relevance Pistachio_ringbreaker, Template_relevance Reaxys, Template_relevance Reaxys_biocatalysis model, leveraging a vast database of chemical reactions to predict feasible synthetic routes.
One-Step Synthesis Focus: Specifically designed for one-step synthesis, it provides concise and direct routes for your target compounds, streamlining the synthesis process.
Accurate Predictions: Utilizing the extensive PISTACHIO, BKMS_METABOLIC, PISTACHIO_RINGBREAKER, REAXYS, REAXYS_BIOCATALYSIS database, our tool offers high-accuracy predictions, reflecting the latest in chemical research and data.
Strategy Settings
Precursor scoring | Relevance Heuristic |
---|---|
Min. plausibility | 0.01 |
Model | Template_relevance |
Template Set | Pistachio/Bkms_metabolic/Pistachio_ringbreaker/Reaxys/Reaxys_biocatalysis |
Top-N result to add to graph | 6 |
Feasible Synthetic Routes
体外研究产品的免责声明和信息
请注意,BenchChem 上展示的所有文章和产品信息仅供信息参考。 BenchChem 上可购买的产品专为体外研究设计,这些研究在生物体外进行。体外研究,源自拉丁语 "in glass",涉及在受控实验室环境中使用细胞或组织进行的实验。重要的是要注意,这些产品没有被归类为药物或药品,他们没有得到 FDA 的批准,用于预防、治疗或治愈任何医疗状况、疾病或疾病。我们必须强调,将这些产品以任何形式引入人类或动物的身体都是法律严格禁止的。遵守这些指南对确保研究和实验的法律和道德标准的符合性至关重要。