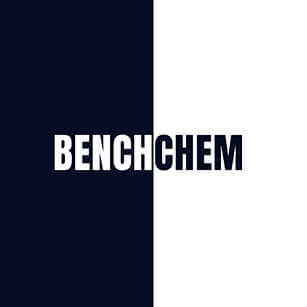
Ceftibuten Related Impurity 6
- 点击 快速询问 获取最新报价。
- 提供有竞争力价格的高质量产品,您可以更专注于研究。
描述
Ceftibuten Related Impurity 6 is a chemical compound associated with the antibiotic ceftibuten, a third-generation cephalosporin. Ceftibuten is used to treat various bacterial infections, including acute bacterial exacerbations of chronic bronchitis, acute bacterial otitis media, pharyngitis, and tonsillitis . Impurities like this compound are often studied to ensure the purity and efficacy of pharmaceutical products.
准备方法
The synthesis of Ceftibuten Related Impurity 6 involves multiple steps, including acylation, condensation, and hydrolysis. Two primary methods are used for the synthesis of the 7β side chain of ceftibuten:
Method 1: The target compounds are prepared from methyl 2-(2-aminothiazol-4-yl) acetate by acylation, condensation, and hydrolysis.
Method 2: The target compounds are prepared from phenylmethyl 2-(2-acylated aminothiazol-4-yl) acetate by condensation, Weiss-Titin reaction, and hydrolysis.
化学反应分析
Ceftibuten Related Impurity 6 undergoes various chemical reactions, including:
Oxidation: This reaction involves the addition of oxygen or the removal of hydrogen. Common oxidizing agents include potassium permanganate and hydrogen peroxide.
Reduction: This reaction involves the addition of hydrogen or the removal of oxygen. Common reducing agents include lithium aluminum hydride and sodium borohydride.
Substitution: This reaction involves the replacement of one atom or group of atoms with another. Common reagents include halogens and nucleophiles.
The major products formed from these reactions depend on the specific conditions and reagents used.
科学研究应用
Ceftibuten Related Impurity 6 has several scientific research applications, including:
Pharmaceutical Research: It is used in the development and validation of analytical methods for ceftibuten and its impurities.
Quality Control: It is used in quality control processes to ensure the purity and efficacy of ceftibuten products.
Stability Studies: It is used in stability studies to assess the shelf life and stability of ceftibuten products.
Genotoxic Potential Assessment: It is used to identify unknown impurities and assess their genotoxic potential.
作用机制
Ceftibuten Related Impurity 6, like ceftibuten, exerts its effects by inhibiting bacterial cell wall synthesis. It binds to one or more penicillin-binding proteins (PBPs), which inhibits the final transpeptidation step of peptidoglycan synthesis in bacterial cell walls . This inhibition leads to the cessation of cell wall biosynthesis, ultimately causing bacterial cell lysis due to the activity of cell wall autolysis enzymes .
相似化合物的比较
Ceftibuten Related Impurity 6 can be compared with other cephalosporin-related impurities, such as:
Ceftibuten Related Impurity 1: This impurity has a different molecular structure and may exhibit different chemical properties.
Ceftibuten Related Impurity 3: This impurity also differs in its molecular structure and chemical properties.
Ceftibuten Related Impurity 4: Similar to the other impurities, it has unique chemical properties and potential effects.
This compound is unique due to its specific molecular structure and the particular synthetic routes used for its preparation.
属性
CAS 编号 |
57028-71-4 |
---|---|
分子式 |
C20H18N2O3S |
分子量 |
366.4 g/mol |
IUPAC 名称 |
benzhydryl (6R,7R)-7-amino-8-oxo-5-thia-1-azabicyclo[4.2.0]oct-3-ene-2-carboxylate |
InChI |
InChI=1S/C20H18N2O3S/c21-16-18(23)22-15(11-12-26-19(16)22)20(24)25-17(13-7-3-1-4-8-13)14-9-5-2-6-10-14/h1-12,15-17,19H,21H2/t15?,16-,19-/m1/s1 |
InChI 键 |
ODKAYLSZYDIQRV-HFLHWUEFSA-N |
手性 SMILES |
C1=CC=C(C=C1)C(C2=CC=CC=C2)OC(=O)C3C=CS[C@H]4N3C(=O)[C@H]4N |
规范 SMILES |
C1=CC=C(C=C1)C(C2=CC=CC=C2)OC(=O)C3C=CSC4N3C(=O)C4N |
纯度 |
> 95% |
数量 |
Milligrams-Grams |
同义词 |
5-Thia-1-azabicyclo[4.2.0]oct-3-ene-2-carboxylic acid, 7-amino-8-oxo-, diphenylmethyl ester, [6R-(6α,7β)]- (9CI) |
产品来源 |
United States |
体外研究产品的免责声明和信息
请注意,BenchChem 上展示的所有文章和产品信息仅供信息参考。 BenchChem 上可购买的产品专为体外研究设计,这些研究在生物体外进行。体外研究,源自拉丁语 "in glass",涉及在受控实验室环境中使用细胞或组织进行的实验。重要的是要注意,这些产品没有被归类为药物或药品,他们没有得到 FDA 的批准,用于预防、治疗或治愈任何医疗状况、疾病或疾病。我们必须强调,将这些产品以任何形式引入人类或动物的身体都是法律严格禁止的。遵守这些指南对确保研究和实验的法律和道德标准的符合性至关重要。