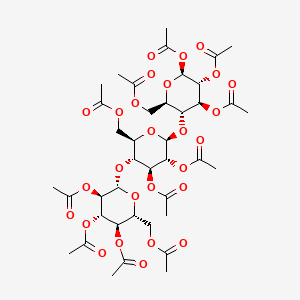
醋酸纤维素
描述
Triacetylcellulose is a chemical compound derived from cellulose, a natural polymer found in the cell walls of plants. It is produced by acetylating cellulose with acetic acid and/or acetic anhydride, converting the hydroxyl groups in cellulose to acetyl groups. This modification renders the cellulose polymer much more soluble in organic solvents. Triacetylcellulose is commonly used for the creation of fibers and film bases and is chemically similar to cellulose acetate. Its distinguishing characteristic is that at least 92 percent of the hydroxyl groups are acetylated .
科学研究应用
Triacetylcellulose has a wide range of applications in scientific research and industry:
Membranes: Used in the fabrication of porous membranes for filtration and separation processes.
Optical Devices: Utilized in the production of polarized films for liquid crystal displays.
Biocomposites: Employed in the development of biodegradable and “all cellulose” biocomposite systems.
Textiles: Used in the production of wrinkle-resistant and shrink-resistant fabrics.
作用机制
Target of Action
Cellulose triacetate (CTA) is a chemical compound derived from cellulose, a natural polymer. The primary target of CTA is cellulose itself . The process involves the acetylation of cellulose, converting hydroxyl groups in cellulose to acetyl groups . This modification enhances the solubility of the cellulose polymer in organic solvents .
Mode of Action
The mode of action of CTA involves a chemical reaction known as acetylation. In this process, cellulose is acetylated with acetic acid and/or acetic anhydride . This acetylation converts hydroxyl groups in cellulose to acetyl groups, rendering the cellulose polymer much more soluble in organic solvents . The cellulose acetate is then dissolved in a mixture of dichloromethane and methanol for spinning .
Biochemical Pathways
The biochemical pathway involved in the action of CTA is the acetylation of cellulose. This process converts the hydroxyl groups in cellulose to acetyl groups, which significantly enhances the solubility of the cellulose polymer in organic solvents . The acetylation of cellulose is a key step in the production of CTA .
Pharmacokinetics
For instance, in hemodialysis, the properties of CTA can affect the clearance of certain drugs like vancomycin .
Result of Action
The result of the acetylation process is the formation of CTA, a material that is more heat resistant than cellulose acetate . CTA is commonly used for the creation of fibers and film base . It is particularly effective in clothing where crease or pleat retention is important .
Action Environment
The action environment can influence the action, efficacy, and stability of CTA. For instance, the synthesis of CTA can be influenced by the choice of solvent, temperature, and other conditions . Moreover, environmental factors can also influence the properties of the resulting CTA material .
生化分析
Biochemical Properties
Cellulose triacetate has been synthesized from corn stover cellulose (CSC) in the presence of [PyPS]3PW12O40 (IL-POM) . The effects of IL-POM contents, reaction temperature, and reaction time on the yield and degree of substitution of cellulose triacetate were investigated .
Cellular Effects
The biocompatibility of cellulose triacetate has been studied in the context of dialysis membranes . The physical structure of the membrane, whether homogeneous or asymmetric, does not significantly affect its biocompatibility .
Molecular Mechanism
The molecular mechanism of cellulose triacetate involves the acetylation of cellulose, which increases its solubility in organic solvents . This property is crucial for its use in various applications, including the manufacture of dialysis membranes .
Temporal Effects in Laboratory Settings
In laboratory settings, the stability and degradation of cellulose triacetate are important factors to consider. The decomposition temperature of cellulose triacetate increases by about 30 °C compared to that of corn stover cellulose .
Metabolic Pathways
Cellulose triacetate is involved in the acetylation pathway, where cellulose is converted into an acetylated derivative
Transport and Distribution
The transport and distribution of cellulose triacetate within cells and tissues are largely dependent on its solubility in organic solvents
Subcellular Localization
Given its solubility properties, it is likely to be found in areas of the cell where organic solvents are present
准备方法
Synthetic Routes and Reaction Conditions
Triacetylcellulose is synthesized through the acetylation of cellulose. The process involves reacting cellulose with acetic anhydride in the presence of a catalyst, typically sulfuric acid. The reaction conditions include maintaining a temperature of around 50-60°C and stirring the mixture for several hours to ensure complete acetylation. The degree of substitution, which indicates the number of hydroxyl groups replaced by acetyl groups, is typically around 2.9 .
Industrial Production Methods
In industrial settings, cellulose triacetate is produced using a similar acetylation process. The cellulose is dissolved in a mixture of dichloromethane and methanol, and the acetylation reaction is carried out in large reactors. The resulting cellulose triacetate is then precipitated, washed, and dried. The fibers are spun using a dry spinning process, where the solvent is evaporated in warm air, leaving behind almost pure cellulose triacetate fibers .
化学反应分析
Types of Reactions
Triacetylcellulose undergoes various chemical reactions, including:
Hydrolysis: The acetyl groups can be hydrolyzed back to hydroxyl groups in the presence of water and an acid or base catalyst.
Saponification: The acetyl groups can be removed using a sodium hydroxide solution, leaving a cellulose coating on the fibers.
Common Reagents and Conditions
Hydrolysis: Water, acid or base catalyst (e.g., sulfuric acid or sodium hydroxide).
Saponification: Sodium hydroxide solution.
Major Products Formed
Hydrolysis: Cellulose and acetic acid.
Saponification: Cellulose and sodium acetate.
相似化合物的比较
Triacetylcellulose is often compared with other cellulose derivatives, such as cellulose acetate and cellulose diacetate. The key differences include:
Degree of Acetylation: Triacetylcellulose has a higher degree of acetylation (at least 92 percent) compared to cellulose acetate and cellulose diacetate.
Thermal Stability: Triacetylcellulose is more heat resistant than cellulose acetate.
Solubility: Triacetylcellulose is more soluble in organic solvents due to the higher degree of acetylation.
Similar Compounds
- Cellulose Acetate
- Cellulose Diacetate
Triacetylcellulose’s unique properties, such as high thermal stability and solubility, make it a valuable compound in various applications, distinguishing it from other cellulose derivatives.
属性
IUPAC Name |
[(2R,3R,4S,5R,6S)-4,5-diacetyloxy-3-[(2S,3R,4S,5R,6R)-3,4,5-triacetyloxy-6-(acetyloxymethyl)oxan-2-yl]oxy-6-[(2R,3R,4S,5R,6S)-4,5,6-triacetyloxy-2-(acetyloxymethyl)oxan-3-yl]oxyoxan-2-yl]methyl acetate | |
---|---|---|
Source | PubChem | |
URL | https://pubchem.ncbi.nlm.nih.gov | |
Description | Data deposited in or computed by PubChem | |
InChI |
InChI=1S/C40H54O27/c1-15(41)52-12-26-29(55-18(4)44)32(56-19(5)45)36(60-23(9)49)39(64-26)67-31-28(14-54-17(3)43)65-40(37(61-24(10)50)34(31)58-21(7)47)66-30-27(13-53-16(2)42)63-38(62-25(11)51)35(59-22(8)48)33(30)57-20(6)46/h26-40H,12-14H2,1-11H3/t26-,27-,28-,29-,30-,31-,32+,33+,34+,35-,36-,37-,38-,39+,40+/m1/s1 | |
Source | PubChem | |
URL | https://pubchem.ncbi.nlm.nih.gov | |
Description | Data deposited in or computed by PubChem | |
InChI Key |
NNLVGZFZQQXQNW-ADJNRHBOSA-N | |
Source | PubChem | |
URL | https://pubchem.ncbi.nlm.nih.gov | |
Description | Data deposited in or computed by PubChem | |
Canonical SMILES |
CC(=O)OCC1C(C(C(C(O1)OC2C(OC(C(C2OC(=O)C)OC(=O)C)OC(=O)C)COC(=O)C)OC(=O)C)OC(=O)C)OC3C(C(C(C(O3)COC(=O)C)OC(=O)C)OC(=O)C)OC(=O)C | |
Source | PubChem | |
URL | https://pubchem.ncbi.nlm.nih.gov | |
Description | Data deposited in or computed by PubChem | |
Isomeric SMILES |
CC(=O)OC[C@@H]1[C@H]([C@@H]([C@H]([C@@H](O1)O[C@@H]2[C@H](O[C@H]([C@@H]([C@H]2OC(=O)C)OC(=O)C)OC(=O)C)COC(=O)C)OC(=O)C)OC(=O)C)O[C@H]3[C@@H]([C@H]([C@@H]([C@H](O3)COC(=O)C)OC(=O)C)OC(=O)C)OC(=O)C | |
Source | PubChem | |
URL | https://pubchem.ncbi.nlm.nih.gov | |
Description | Data deposited in or computed by PubChem | |
Molecular Formula |
C40H54O27 | |
Source | PubChem | |
URL | https://pubchem.ncbi.nlm.nih.gov | |
Description | Data deposited in or computed by PubChem | |
Molecular Weight |
966.8 g/mol | |
Source | PubChem | |
URL | https://pubchem.ncbi.nlm.nih.gov | |
Description | Data deposited in or computed by PubChem | |
CAS No. |
9012-09-3 | |
Record name | Cellulose, triacetate | |
Source | EPA Chemicals under the TSCA | |
URL | https://www.epa.gov/chemicals-under-tsca | |
Description | EPA Chemicals under the Toxic Substances Control Act (TSCA) collection contains information on chemicals and their regulations under TSCA, including non-confidential content from the TSCA Chemical Substance Inventory and Chemical Data Reporting. | |
Record name | Cellulose, triacetate | |
Source | European Chemicals Agency (ECHA) | |
URL | https://echa.europa.eu/substance-information/-/substanceinfo/100.115.198 | |
Description | The European Chemicals Agency (ECHA) is an agency of the European Union which is the driving force among regulatory authorities in implementing the EU's groundbreaking chemicals legislation for the benefit of human health and the environment as well as for innovation and competitiveness. | |
Explanation | Use of the information, documents and data from the ECHA website is subject to the terms and conditions of this Legal Notice, and subject to other binding limitations provided for under applicable law, the information, documents and data made available on the ECHA website may be reproduced, distributed and/or used, totally or in part, for non-commercial purposes provided that ECHA is acknowledged as the source: "Source: European Chemicals Agency, http://echa.europa.eu/". Such acknowledgement must be included in each copy of the material. ECHA permits and encourages organisations and individuals to create links to the ECHA website under the following cumulative conditions: Links can only be made to webpages that provide a link to the Legal Notice page. | |
Record name | Cellulose triacetate | |
Source | Human Metabolome Database (HMDB) | |
URL | http://www.hmdb.ca/metabolites/HMDB0032198 | |
Description | The Human Metabolome Database (HMDB) is a freely available electronic database containing detailed information about small molecule metabolites found in the human body. | |
Explanation | HMDB is offered to the public as a freely available resource. Use and re-distribution of the data, in whole or in part, for commercial purposes requires explicit permission of the authors and explicit acknowledgment of the source material (HMDB) and the original publication (see the HMDB citing page). We ask that users who download significant portions of the database cite the HMDB paper in any resulting publications. | |
体外研究产品的免责声明和信息
请注意,BenchChem 上展示的所有文章和产品信息仅供信息参考。 BenchChem 上可购买的产品专为体外研究设计,这些研究在生物体外进行。体外研究,源自拉丁语 "in glass",涉及在受控实验室环境中使用细胞或组织进行的实验。重要的是要注意,这些产品没有被归类为药物或药品,他们没有得到 FDA 的批准,用于预防、治疗或治愈任何医疗状况、疾病或疾病。我们必须强调,将这些产品以任何形式引入人类或动物的身体都是法律严格禁止的。遵守这些指南对确保研究和实验的法律和道德标准的符合性至关重要。