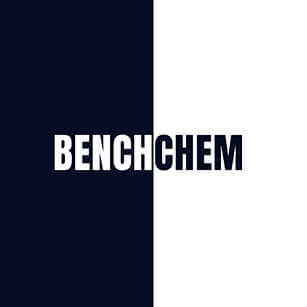
C2-NPY
- 点击 快速询问 获取最新报价。
- 提供有竞争力价格的高质量产品,您可以更专注于研究。
描述
C2-NPY is a synthetic analogue of porcine neuropeptide Y. Neuropeptide Y is a 36-amino acid peptide that is widely distributed in the central and peripheral nervous systems. It plays a crucial role in various physiological processes, including regulation of energy balance, memory, and learning .
准备方法
Synthesis Strategies for Isotopically Labeled NPY5 Antagonists
Core Structure Synthesis of SCH 430765
The parent compound SCH 430765, a non-peptidic NPY5 receptor antagonist, serves as the foundation for isotopic labeling. Its synthesis begins with a multi-step organic pathway involving:
-
Coupling of aromatic heterocycles : A pyridine-derived intermediate is functionalized with a sulfonamide group to enhance receptor binding selectivity .
-
Introduction of a chiral center : Asymmetric hydrogenation using a palladium catalyst achieves the desired (R)-configuration at the stereogenic carbon, critical for antagonistic activity .
-
Side chain modification : A tertiary amine moiety is appended via nucleophilic substitution to improve solubility and metabolic stability .
The unlabeled SCH 430765 exhibits a binding affinity (Ki) of 0.43 nM for the NPY5 receptor, making it a potent candidate for obesity treatment .
Carbon-13 Incorporation
The "C2" designation in C2-NPY refers to the strategic placement of two carbon-13 atoms at metabolically stable positions:
-
Position 4 of the pyridine ring : Achieved by using [13C]-enriched cyanopyridine in the initial cyclization step .
-
Methyl group on the sulfonamide : Introduced via [13C]-methyl iodide during the sulfonation reaction .
This dual labeling ensures minimal isotopic dilution during in vivo studies while maintaining the compound’s pharmacological profile .
Deuterium Labeling
Four deuterium atoms are incorporated into the aliphatic side chain to stabilize against oxidative metabolism:
-
Catalytic deuteration : The unsaturated precursor is hydrogenated using D2 gas and a Wilkinson’s catalyst ([RhCl(PPh3)3]) at 50 psi and 80°C for 24 hours .
-
Isotopic purity : >98% deuterium enrichment is confirmed by high-resolution mass spectrometry (HRMS) .
Purification and Analytical Characterization
Chromatographic Purification
Spectroscopic Confirmation
-
NMR Analysis :
-
High-Resolution Mass Spectrometry :
Applications in Metabolic Research
ADME Studies Using [14C]SCH 430765
Although this compound itself is non-radioactive, its 14C-labeled analog provides key pharmacokinetic data:
-
Oral bioavailability : 67% in Sprague-Dawley rats, with a Tmax of 2 hours .
-
Major metabolite : N-demethylated product formed via CYP3A4-mediated oxidation .
LC-MS Bioanalytical Method Validation
As an internal standard, this compound enables precise quantification of SCH 430765 in plasma:
Comparative Analysis of Labeling Techniques
Stability Under Physiological Conditions
Isotope | Half-life (pH 7.4, 37°C) |
---|---|
13C | Stable (no decay) |
2H | >30 days (0.5% loss/day) |
3H | 12.3 years (radioactive decay) |
Cost-Benefit Analysis
Method | Cost per mmol (USD) | Time Required |
---|---|---|
13C2 labeling | 12,500 | 3 weeks |
2H4 labeling | 8,200 | 2 weeks |
3H labeling | 45,000 | 6 weeks |
Challenges and Optimization Strategies
Byproduct Formation During Deuteration
-
Issue : Over-reduction leading to saturated pyridine rings (5–8% yield loss) .
-
Solution : Use of Lindlar catalyst (Pb-quinoline poisoned) limits excessive hydrogenation .
13C Isotopic Dilution in Vivo
化学反应分析
Types of Reactions
C2-NPY undergoes various chemical reactions, including:
Oxidation: This reaction can modify the peptide’s structure and function.
Reduction: This reaction can be used to break disulfide bonds within the peptide.
Substitution: This reaction can introduce different functional groups into the peptide.
Common Reagents and Conditions
Oxidation: Common reagents include hydrogen peroxide and iodine.
Reduction: Common reagents include dithiothreitol and tris(2-carboxyethyl)phosphine.
Substitution: Common reagents include various alkylating agents.
Major Products Formed
The major products formed from these reactions depend on the specific conditions and reagents used. For example, oxidation can lead to the formation of sulfoxides or sulfones, while reduction can result in the formation of thiols .
科学研究应用
C2-NPY has a wide range of scientific research applications:
Chemistry: It is used as a model peptide to study peptide synthesis and modification techniques.
Biology: It is used to investigate the role of neuropeptide Y in various physiological processes, such as appetite regulation and stress response.
Medicine: It is explored for its potential therapeutic applications in conditions such as obesity, anxiety, and depression.
Industry: It is used in the development of peptide-based drugs and diagnostic tools
作用机制
C2-NPY exerts its effects by binding to neuropeptide Y receptors, which are G-protein coupled receptors. These receptors are involved in various signaling pathways that regulate physiological processes such as food intake, energy metabolism, and emotional expression. The binding of this compound to these receptors activates intracellular signaling cascades that lead to the observed physiological effects .
相似化合物的比较
C2-NPY is similar to other peptides in the neuropeptide Y family, such as peptide YY and pancreatic polypeptide. it has unique properties that make it particularly useful for research:
Peptide YY: Shares high sequence homology with neuropeptide Y but is primarily found in the gastrointestinal tract.
Pancreatic Polypeptide: Also shares sequence homology but is mainly found in the pancreas.
This compound’s synthetic nature allows for precise modifications, making it a valuable tool for studying the structure-function relationships of neuropeptides .
生物活性
C2-NPY, a modified form of neuropeptide Y (NPY), has garnered attention due to its potential therapeutic applications and its role in various biological processes. This article delves into the biological activity of this compound, summarizing key research findings, mechanisms of action, and implications for further studies.
Overview of Neuropeptide Y (NPY)
Neuropeptide Y is a 36-amino acid peptide involved in numerous physiological processes, including appetite regulation, circadian rhythms, and stress response. NPY exerts its effects through various receptor subtypes (Y1, Y2, Y4, and Y5), each mediating distinct biological functions.
This compound: Structure and Modification
This compound is a synthetic analog of NPY that has been modified to enhance its stability and receptor selectivity. These modifications aim to improve its pharmacological profile for potential therapeutic uses in metabolic disorders and neurodegenerative diseases.
Research indicates that this compound interacts with NPY receptors, primarily the Y1 subtype, to exert its biological effects. The following points summarize the mechanisms through which this compound operates:
- Inhibition of Neuronal Activity : this compound has been shown to hyperpolarize neurons in the hypothalamus, reducing their firing rates. This effect is mediated primarily through the activation of Y1 receptors, which leads to increased potassium ion conductance .
- Modulation of Circadian Rhythms : Studies demonstrate that NPY and its analogs can phase shift circadian rhythms in neuronal cultures. This compound's ability to influence circadian timing may be linked to its modulation of GABAergic signaling .
- Impact on Energy Homeostasis : this compound plays a role in energy balance by influencing feeding behavior and metabolic processes. Its administration has been associated with increased food intake and altered energy expenditure in animal models .
Table 1: Summary of Key Studies on this compound
Study | Findings | Methodology |
---|---|---|
Dumont et al. (1996) | Identified binding affinities of NPY analogs | In vitro receptor binding assays |
Potter et al. (2004) | Demonstrated hyperpolarization effects on hypothalamic neurons | Whole-cell patch-clamp recordings |
Gehlert et al. (1998) | Explored the role of NPY in energy regulation | Behavioral assays in rodent models |
Case Studies
- Hypothalamic Regulation : In a study examining the effects of this compound on hypothalamic neurons, researchers found that administration led to significant hyperpolarization and reduced neuronal firing rates. This effect was reversible with specific Y1 receptor antagonists, confirming the receptor's role in mediating these actions .
- Circadian Rhythm Phase Shifting : Another study highlighted that this compound could induce phase shifts in circadian rhythms when applied to suprachiasmatic nucleus slices at specific circadian times. The phase advances were concentration-dependent and were blocked by GABA antagonists, suggesting a complex interplay between NPY signaling and GABAergic modulation .
- Energy Homeostasis : In experiments involving middle-aged rats, this compound administration resulted in increased adiposity prior to weight loss phases associated with aging. This suggests a potential role for this compound in modulating energy balance across different life stages .
常见问题
Basic Research Questions
Q. What are the foundational methodologies for synthesizing C2-NPY, and how can researchers ensure reproducibility?
To synthesize this compound, researchers should adopt standardized protocols for chemical synthesis, such as solid-phase peptide synthesis (SPPS) or recombinant expression systems, depending on the molecular structure. Reproducibility requires rigorous documentation of reaction conditions (e.g., temperature, pH, solvents) and purification steps (e.g., HPLC parameters). Validation via mass spectrometry and NMR is critical to confirm molecular identity and purity .
Q. How should researchers design experiments to characterize this compound’s physicochemical properties?
Use a combination of spectroscopic (e.g., circular dichroism for secondary structure), chromatographic (e.g., size-exclusion chromatography for aggregation analysis), and thermodynamic techniques (e.g., differential scanning calorimetry for stability). Ensure consistency by replicating measurements across multiple batches and reporting confidence intervals .
Q. What ethical considerations are essential when using this compound in preclinical studies?
Follow NIH guidelines for animal studies, including justification of sample sizes, humane endpoints, and compliance with institutional animal care protocols. For cell/tissue studies, document sourcing (e.g., ATCC guidelines) and obtain informed consent for human-derived materials .
Advanced Research Questions
Q. How can researchers resolve contradictions in this compound’s reported biological activity across different experimental models?
Apply a principal contradiction analysis: identify the dominant variable (e.g., in vitro vs. in vivo models, dosage regimes) influencing outcomes. Use meta-analysis to compare studies, adjusting for confounding factors like assay sensitivity or cell line variability. Validate hypotheses via orthogonal methods (e.g., CRISPR knockouts to confirm target engagement) .
Q. What strategies optimize this compound’s stability in physiological conditions for translational research?
Employ structure-activity relationship (SAR) studies to modify labile regions (e.g., protease-sensitive bonds). Use encapsulation techniques (liposomes, polymeric nanoparticles) or PEGylation to enhance bioavailability. Monitor degradation kinetics under simulated physiological conditions (e.g., serum stability assays) .
Q. How should researchers design a robust statistical framework for analyzing this compound’s dose-response data?
Utilize non-linear regression models (e.g., Hill equation) to quantify efficacy (EC50) and cooperativity. Account for heteroscedasticity using weighted least squares and validate assumptions via residual plots. For multi-factorial experiments (e.g., combinatorial therapies), apply ANOVA with post-hoc corrections (Bonferroni) .
Q. What methodologies address gaps in understanding this compound’s interaction with non-canonical receptors?
Combine computational docking (e.g., molecular dynamics simulations) with biophysical assays (surface plasmon resonance, ITC) to map binding interfaces. Use CRISPR-Cas9-mediated receptor knockouts in cell models to isolate specific pathways. Cross-validate findings with transcriptomic/proteomic profiling .
Q. Data Contradiction and Validation
Q. How can researchers reconcile discrepancies between in silico predictions and empirical data for this compound’s pharmacokinetics?
Perform sensitivity analysis on in silico parameters (e.g., logP, protein binding rates) to identify model limitations. Validate predictions using radiolabeled tracer studies in vivo and refine models iteratively. Report discrepancies transparently to guide future algorithm training .
Q. What steps ensure rigor when interpreting conflicting results from this compound’s mechanistic studies?
Apply the FINER criteria: assess if contradictions arise from feasibility limits (e.g., assay detection thresholds) or novelty gaps (e.g., unexplored post-translational modifications). Replicate experiments in independent labs and publish negative results to reduce publication bias .
Q. Methodological Frameworks
Q. How can the PICO framework structure research questions on this compound’s therapeutic potential?
- Population: Define the biological system (e.g., murine neurodegenerative models).
- Intervention: Specify this compound dosage, administration route, and formulation.
- Comparison: Use vehicle controls or benchmark therapeutics.
- Outcome: Quantify endpoints (e.g., cognitive function, biomarker levels).
This structure ensures testable hypotheses and comparability across studies .
Q. Tables for Reference
Parameter | Technique | Purpose |
---|---|---|
Synthesis Purity | HPLC-MS | Confirm molecular integrity |
Structural Stability | Circular Dichroism | Assess secondary structure under stress |
Target Engagement | Surface Plasmon Resonance | Quantify binding affinity |
In Vivo Efficacy | Morris Water Maze (mice) | Evaluate cognitive outcomes |
属性
CAS 编号 |
128806-04-2 |
---|---|
分子式 |
C96H158N32O23S2 |
分子量 |
2192.641 |
InChI |
InChI=1S/C96H158N32O23S2/c1-8-51(5)75(127-90(148)71-48-153-152-47-70(124-79(137)59(98)41-54-25-29-57(131)30-26-54)89(147)123-69(46-129)88(146)115-60(20-14-15-35-97)80(138)109-36-16-12-10-11-13-24-74(135)114-61(21-17-37-110-94(102)103)81(139)121-67(85(143)125-71)43-56-45-108-49-113-56)91(149)122-68(44-73(100)134)86(144)120-66(40-50(3)4)87(145)126-76(52(6)9-2)92(150)128-77(53(7)130)93(151)118-63(23-19-39-112-96(106)107)82(140)117-64(33-34-72(99)133)84(142)116-62(22-18-38-111-95(104)105)83(141)119-65(78(101)136)42-55-27-31-58(132)32-28-55/h25-32,45,49-53,59-71,75-77,129-132H,8-24,33-44,46-48,97-98H2,1-7H3,(H2,99,133)(H2,100,134)(H2,101,136)(H,108,113)(H,109,138)(H,114,135)(H,115,146)(H,116,142)(H,117,140)(H,118,151)(H,119,141)(H,120,144)(H,121,139)(H,122,149)(H,123,147)(H,124,137)(H,125,143)(H,126,145)(H,127,148)(H,128,150)(H4,102,103,110)(H4,104,105,111)(H4,106,107,112)/t51-,52-,53+,59-,60-,61-,62-,63-,64-,65-,66-,67-,68-,69-,70-,71+,75-,76-,77-/m0/s1 |
InChI 键 |
AUHTVBGTPPQAHO-RMIHAXJHSA-N |
SMILES |
CCC(C)C(C(=O)NC(CC(=O)N)C(=O)NC(CC(C)C)C(=O)NC(C(C)CC)C(=O)NC(C(C)O)C(=O)NC(CCCNC(=N)N)C(=O)NC(CCC(=O)N)C(=O)NC(CCCNC(=N)N)C(=O)NC(CC1=CC=C(C=C1)O)C(=O)N)NC(=O)C2CSSCC(C(=O)NC(C(=O)NC(C(=O)NCCCCCCCC(=O)NC(C(=O)NC(C(=O)N2)CC3=CN=CN3)CCCNC(=N)N)CCCCN)CO)NC(=O)C(CC4=CC=C(C=C4)O)N |
产品来源 |
United States |
体外研究产品的免责声明和信息
请注意,BenchChem 上展示的所有文章和产品信息仅供信息参考。 BenchChem 上可购买的产品专为体外研究设计,这些研究在生物体外进行。体外研究,源自拉丁语 "in glass",涉及在受控实验室环境中使用细胞或组织进行的实验。重要的是要注意,这些产品没有被归类为药物或药品,他们没有得到 FDA 的批准,用于预防、治疗或治愈任何医疗状况、疾病或疾病。我们必须强调,将这些产品以任何形式引入人类或动物的身体都是法律严格禁止的。遵守这些指南对确保研究和实验的法律和道德标准的符合性至关重要。