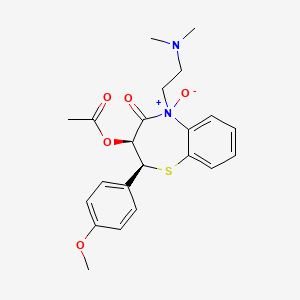
Diltiazem N-oxide
描述
Diltiazem N-oxide (CAS 142843-04-7) is a major metabolite of diltiazem, a benzothiazepine-class calcium channel blocker used clinically for hypertension and angina. Structurally, it is characterized by the oxidation of the dimethylamino group in diltiazem to an N-oxide moiety. Its molecular formula is C22H25N2O6S<sup>+</sup>, with a molecular weight of 453.51 g/mol. The SMILES notation is COC1=CC=C(C=C1)[C@@H](SC2=C3C=CC(=O)N=C3N(CCO)C2=O)OC(=O)C.[O-], and its InChIKey is VZTVRIXPYNLDQN-QXBGKQBOSA-M .
This compound is synthesized via hepatic metabolism of diltiazem, alongside other metabolites such as O-demethyl diltiazem and N-desmethyl diltiazem .
准备方法
Chemical Context and Significance of Diltiazem N-Oxide
This compound arises during the asymmetric dihydroxylation (AD) step in diltiazem synthesis, where nitrogen oxidation plays a pivotal role in generating chiral intermediates . The compound’s preparation is intricately linked to the use of trifunctional heterogeneous catalysts and recyclable ion-exchange systems, which enable high enantiomeric purity (>99%) while eliminating toxic osmium residues . Its formation is a prerequisite for subsequent cyclization and acylation steps that yield the final active pharmaceutical ingredient (API).
Catalytic N-Oxidation in Diltiazem Synthesis
Role of N-Oxidation in Asymmetric Dihydroxylation
The N-oxidation step involves converting tertiary amines (e.g., N-methylmorpholine, trimethylamine) into N-oxides using hydrogen peroxide (H₂O₂) as a terminal oxidant . This reaction generates N-methylmorpholine N-oxide (NMO), which serves as a co-oxidant in the osmium-catalyzed dihydroxylation of ethyl acrylate and 4-iodoanisole. The process occurs in a single pot using recyclable ion-exchanger-supported palladium-osmium-tungsten (IE-PdOsW) catalysts, which simultaneously mediate Heck coupling and asymmetric dihydroxylation .
Key Conditions :
-
Catalysts : LDH-PdOsW, SiO₂-PdOsW, or resin-PdOsW (0.1–1 mol%)
-
Solvents : Water, acetone, acetonitrile, or tert-butanol
-
Temperature : 70°C for Heck coupling; ambient for N-oxidation
-
Yield : 85–98% for Heck coupling; quantitative NMO generation
Mechanistic Insights
The IE-PdOsW catalyst facilitates three concurrent reactions:
-
Heck Coupling : Pd⁰-mediated coupling of 4-iodoanisole and ethyl acrylate to form prochiral olefins.
-
N-Oxidation : H₂O₂ oxidizes N-methylmorpholine to NMO, regenerating Os⁸⁺ for asymmetric dihydroxylation.
-
Asymmetric Dihydroxylation : OsO₄ (generated in situ) and chiral ligands (e.g., (DHQ)₂PHAL) induce enantioselective dihydroxylation .
This trifunctional mechanism eliminates the need for external OsO₄, reducing toxicity and cost .
Synthetic Protocols for this compound
Heterogeneous Catalysis Approach
A patent-pending method (WO2004058734A1) outlines the following optimized protocol :
Step 1: Synthesis of (2R,3S)-(-)-Ethyl-2,3-dihydroxy-3-(4-methoxyphenyl)propionate
-
Reactants : 4-iodoanisole (10 mmol), ethyl acrylate (10 mmol), triethylamine (11 mmol)
-
Catalyst : LDH-PdOsW (0.4 g, 0.1 mmol)
-
Conditions : 70°C, 16 h, N₂ atmosphere
Step 2: N-Oxidation and Asymmetric Dihydroxylation
-
Oxidant : H₂O₂ (2 equiv)
-
Amine : N-methylmorpholine (NMM, 1.2 equiv)
-
Chiral Ligand : (DHQ)₂PHAL (1 mol%)
-
Solvent : tert-Butanol/water (3:1)
Step 3: Cyclic Sulfite Formation and Lactamization
-
Sulfitation : Thionyl chloride (1.3 equiv) in pyridine at 0°C for 2 h.
-
Lactamization : Fe³⁺-exchanged clay catalyzes reaction with 2-aminothiophenol in xylene (120–140°C, 12 h) .
Process Optimization and Environmental Considerations
Catalyst Recycling and Waste Reduction
The IE-PdOsW system is recoverable via filtration and reused for 5 cycles without significant activity loss (<5% yield drop) . Fe³⁺-clay in lactamization eliminates acidic wastewater, reducing environmental impact .
Comparative Analysis of N-Oxidants
Oxidant | Solvent | Temperature (°C) | ee (%) | Yield (%) |
---|---|---|---|---|
H₂O₂/NMM | t-BuOH/H₂O | 25 | >99 | 85 |
TBHP/Triethylamine | Acetone | 40 | 95 | 78 |
O₂/CuCl₂ | MeCN | 60 | 88 | 65 |
化学反应分析
Types of Reactions: Diltiazem N-oxide can undergo various chemical reactions, including:
Oxidation: Further oxidation can lead to the formation of more oxidized derivatives.
Reduction: The N-oxide group can be reduced back to the parent amine using reducing agents such as zinc and acetic acid.
Substitution: The N-oxide group can participate in substitution reactions, where it can be replaced by other functional groups under appropriate conditions.
Common Reagents and Conditions:
Oxidation: Hydrogen peroxide, acetic acid.
Reduction: Zinc, acetic acid.
Substitution: Various nucleophiles under acidic or basic conditions.
Major Products:
Oxidation: More oxidized derivatives of this compound.
Reduction: Diltiazem.
Substitution: Substituted derivatives of this compound.
科学研究应用
Diltiazem N-oxide has several applications in scientific research:
Chemistry: Used as a model compound to study the effects of N-oxide functional groups on the chemical and physical properties of molecules.
Biology: Investigated for its potential biological activities, including its effects on calcium channels and other cellular targets.
Medicine: Explored for its potential therapeutic applications, particularly in cardiovascular diseases.
Industry: Used in the development of new pharmaceuticals and as a reference standard in analytical chemistry.
作用机制
The mechanism of action of Diltiazem N-oxide involves its interaction with calcium channels in the cardiovascular system. By inhibiting the influx of calcium ions into cardiac and vascular smooth muscle cells, it helps to relax these muscles, leading to a reduction in blood pressure and alleviation of angina symptoms. The N-oxide group may also influence the binding affinity and selectivity of the compound for its molecular targets.
相似化合物的比较
Diltiazem and Its Metabolites
Diltiazem and its metabolites share a benzothiazepine core but differ in functional groups, leading to variations in pharmacokinetics and pharmacodynamics.
Key Compounds :
Diltiazem : The parent drug acts as a calcium channel antagonist, inhibiting L-type calcium channels with high affinity (KD = 50 nM in skeletal muscle) . It is lipophilic (Class I compound), with unbound concentrations in tissues closely matching plasma levels (Kpuu ≈ 1) .
Diltiazem N-Oxide : The N-oxide metabolite retains the benzothiazepine backbone but has increased polarity due to the oxidized nitrogen. This likely reduces membrane permeability compared to diltiazem, though specific Kpuu data are unavailable.
Deacetyl this compound (CAS 122619-90-3): This derivative lacks the acetyl group of this compound, further altering hydrophobicity. It binds to the same receptors as diltiazem but acts as an agonist rather than an antagonist, suggesting divergent pharmacological effects .
N-Desmethyl Diltiazem : A metabolite contributing to CYP3A4 inactivation, reducing hepatic CYP3A4 levels to 21% of baseline and increasing the AUC of co-administered drugs by 4.7-fold .
Pharmacological Activity :
Structural-Activity Insights :
- Oxidation or demethylation of diltiazem’s amino group reduces calcium channel antagonism. For example, N,O-didemethylated metabolite (IC50 = 112.2 µM) is ~114-fold less potent than diltiazem .
- Deacetylation in this compound may enhance receptor activation, as seen in its agonist activity .
Other N-Oxide Compounds
Antofin N-Oxide : A plant alkaloid from Ficus septica with uncharacterized bioactivity, illustrating the natural occurrence of N-oxides in medicinal plants .
2,6-Dimethylpyridine N-Oxide: Exhibits antimutagenic properties, reducing mutagenesis induced by 2-aminoanthracene and 4-nitroquinoline oxide in bacterial assays .
Contrast with this compound :
- Their N-oxide groups participate in redox reactions or hydrogen bonding, differing from this compound’s role in receptor binding .
生物活性
Diltiazem N-oxide is a metabolite of the calcium channel blocker diltiazem, which is primarily known for its role in treating hypertension and angina. Understanding the biological activity of this compound is crucial for elucidating its pharmacological effects and potential therapeutic applications. This article explores the metabolic pathways, mechanisms of action, and clinical implications associated with this compound.
Metabolism and Formation
Diltiazem undergoes extensive first-pass metabolism, primarily mediated by cytochrome P450 enzymes (CYP3A4 and CYP2D6), resulting in several active metabolites including this compound. The metabolic pathway can be summarized as follows:
- N-demethylation : Diltiazem is metabolized to N-monodesmethyl diltiazem.
- Deacetylation : This metabolite can further be converted to deacetyl diltiazem.
- N-oxidation : Deacetyl diltiazem can undergo N-oxidation to form this compound.
This process is significant as the pharmacological properties of the metabolites can differ from those of the parent compound, influencing their efficacy and safety profiles .
This compound retains some pharmacological activity similar to its parent compound, primarily through its action on calcium channels. It functions as a calcium channel blocker, inhibiting calcium influx into cardiac and smooth muscle cells, leading to:
- Vasodilation : Relaxation of vascular smooth muscle results in decreased blood pressure.
- Reduced myocardial oxygen demand : This effect is particularly beneficial in conditions like angina.
The relative potency of this compound compared to diltiazem has been a subject of investigation, with findings suggesting that while it has reduced activity, it still contributes to the overall therapeutic effects when diltiazem is administered .
Table 1: Comparison of Biological Activities of Diltiazem and this compound
Parameter | Diltiazem | This compound |
---|---|---|
Calcium Channel Blockade | High | Moderate |
Vasodilatory Effect | Strong | Moderate |
Impact on Heart Rate | Decreases | Mild Decrease |
Bioavailability | Low | Low |
Half-Life | 3-5 hours | Variable |
Case Studies
- Coronary Vasospasm Management : A study evaluated the efficacy of diltiazem versus diltiazem combined with nitrates in patients with coronary artery spasm. Results indicated that while both regimens provided relief, there was no significant difference in long-term cardiovascular outcomes between the two treatments .
- Insulin Secretion Studies : Research conducted on rat islets showed that diltiazem influences glucose-induced insulin secretion. The presence of its metabolites, including this compound, was noted to modulate this effect, suggesting potential implications for metabolic regulation .
- Microvascular Dysfunction : In a trial focused on patients with angina and non-obstructive coronary artery disease (ANOCA), diltiazem demonstrated improvements in microvascular function. The role of its metabolites in enhancing coronary flow reserve was highlighted, indicating that this compound may contribute to these beneficial effects .
常见问题
Basic Research Questions
Q. What methods are recommended for characterizing the purity and structure of Diltiazem N-oxide in synthetic chemistry research?
To ensure structural and purity validation, researchers should combine spectroscopic techniques (e.g., NMR, IR) with chromatographic methods. For novel compounds, provide full characterization data, including molecular weight (430.52 g/mol), SMILES notation, and InChIKey (VMYITXJQDGPHNJ-JBAPTLGWSA-N) . For purity, use HPLC with UV detection at 238 nm, ensuring linearity (R² >0.99) and robustness across parameters like flow rate (±0.1 mL/min) and column temperature (±2°C) . Report data in alignment with journal guidelines to avoid redundancy between text and tables .
Q. How can researchers optimize the formulation of this compound using factorial design?
A three-factor, two-level factorial design is effective for pilot-scale optimization. Variables include polymer coating percentage (e.g., hydroxypropyl methylcellulose E15 at 16.66% and 33.33%) and mixing parameters. Analyze results via Pareto charts to identify significant terms (e.g., pilot mix I=24.9%, II=16.6%, III=16.6%) and validate with dissolution testing (USP apparatus 1, 100 rpm, 37°C) . Ensure reproducibility by adhering to strict experimental protocols, such as fluidized bed coating and non-pareil seed preparation .
Q. What HPLC parameters are critical for profiling impurities in this compound?
Key parameters include:
- Column : C18 with gradient elution (e.g., acetonitrile-phosphate buffer).
- Detection : UV at 238 nm.
- Linearity range : 0.3%–150% of impurity tolerance limits.
- Robustness : Test flow rate (±0.2 mL/min), wavelength (±3 nm), and mobile phase composition (±5% organic). Validate using a stability-indicating method to resolve degradants and unknown impurities .
Q. How do dissolution media variations affect this compound release kinetics?
Use a power law equation () to model release from carrageenan matrices. The exponent (estimated via logarithmic linearization) indicates diffusion mechanisms. For example, suggests Fickian diffusion, while indicates Case II transport. Test media with varying pH and ionic strength to simulate physiological conditions .
Q. What are key considerations for ensuring reproducibility in synthesizing this compound derivatives?
- Documentation : Provide detailed synthetic protocols (e.g., reaction time, temperature, purification steps).
- Characterization : Include NMR, HPLC, and mass spectrometry data for novel compounds.
- Purity criteria : Specify residual solvent limits (e.g., DMF <10 ppm) and use alternative solvents where possible .
Advanced Research Questions
Q. What statistical approaches resolve contradictions in dissolution data across experimental conditions?
Apply analysis of variance (ANOVA) with post-hoc Tukey tests to compare release rates under varying conditions (e.g., pH, agitation). Use Pareto charts to prioritize significant factors (e.g., pilot mix ratios) and eliminate non-significant variables. For nonlinear data, employ model simplification techniques validated via goodness-of-fit metrics (e.g., AIC, R² adjusted) .
Q. How does SAR fingerprinting assess the mutagenic potential of aromatic N-oxides like this compound?
SAR fingerprinting involves hierarchical substructure searches (e.g., benzo[c][1,2,5]oxadiazole 1-oxide) to correlate structural motifs with mutagenicity. Use proprietary and public datasets to validate alerts, downgrading general N-oxide alerts but retaining subclasses with strong evidence (e.g., quindioxin). Integrate results into expert-rule-based models like Leadscope for predictive accuracy .
Q. How should researchers design experiments to evaluate Diltiazem’s cardiac effects in post-myocardial infarction models?
Stratify subjects by left ventricular ejection fraction (LVEF) to account for differential outcomes. Use randomized controlled trials (RCTs) with endpoints like mortality and congestive heart failure incidence. For preclinical models, incorporate echocardiography to monitor LV dysfunction and validate findings against clinical data (e.g., MDPIT trial results) .
Q. What methodologies validate stability-indicating analytical methods for this compound?
- Forced degradation : Expose samples to heat, light, and hydrolytic conditions.
- Specificity : Resolve degradants from parent compounds (e.g., deacetyl this compound).
- Robustness : Test variations in column temperature (±5°C) and mobile phase composition. Validate linearity (R² >0.995) and precision (%RSD <2.0) per ICH Q2(R1) guidelines .
Q. What computational tools predict DNA-reactive mutagenicity in N-oxide compounds?
Use (Q)SAR models like Derek Nexus or Leadscope, which incorporate structural alerts for aromatic N-oxides. Validate predictions with Ames test data and proprietary mutagenicity databases. For this compound, focus on subclasses with confirmed alerts (e.g., benzo[c][1,2,5]oxadiazole 1-oxide) and exclude non-mutagenic analogs via SAR fingerprinting .
属性
IUPAC Name |
[(2S,3S)-5-[2-(dimethylamino)ethyl]-2-(4-methoxyphenyl)-5-oxido-4-oxo-2,3-dihydro-1,5-benzothiazepin-5-ium-3-yl] acetate | |
---|---|---|
Source | PubChem | |
URL | https://pubchem.ncbi.nlm.nih.gov | |
Description | Data deposited in or computed by PubChem | |
InChI |
InChI=1S/C22H26N2O5S/c1-15(25)29-20-21(16-9-11-17(28-4)12-10-16)30-19-8-6-5-7-18(19)24(27,22(20)26)14-13-23(2)3/h5-12,20-21H,13-14H2,1-4H3/t20-,21+,24?/m1/s1 | |
Source | PubChem | |
URL | https://pubchem.ncbi.nlm.nih.gov | |
Description | Data deposited in or computed by PubChem | |
InChI Key |
VMYITXJQDGPHNJ-JBAPTLGWSA-N | |
Source | PubChem | |
URL | https://pubchem.ncbi.nlm.nih.gov | |
Description | Data deposited in or computed by PubChem | |
Canonical SMILES |
CC(=O)OC1C(SC2=CC=CC=C2[N+](C1=O)(CCN(C)C)[O-])C3=CC=C(C=C3)OC | |
Source | PubChem | |
URL | https://pubchem.ncbi.nlm.nih.gov | |
Description | Data deposited in or computed by PubChem | |
Isomeric SMILES |
CC(=O)O[C@@H]1[C@@H](SC2=CC=CC=C2[N+](C1=O)(CCN(C)C)[O-])C3=CC=C(C=C3)OC | |
Source | PubChem | |
URL | https://pubchem.ncbi.nlm.nih.gov | |
Description | Data deposited in or computed by PubChem | |
Molecular Formula |
C22H26N2O5S | |
Source | PubChem | |
URL | https://pubchem.ncbi.nlm.nih.gov | |
Description | Data deposited in or computed by PubChem | |
Molecular Weight |
430.5 g/mol | |
Source | PubChem | |
URL | https://pubchem.ncbi.nlm.nih.gov | |
Description | Data deposited in or computed by PubChem | |
体外研究产品的免责声明和信息
请注意,BenchChem 上展示的所有文章和产品信息仅供信息参考。 BenchChem 上可购买的产品专为体外研究设计,这些研究在生物体外进行。体外研究,源自拉丁语 "in glass",涉及在受控实验室环境中使用细胞或组织进行的实验。重要的是要注意,这些产品没有被归类为药物或药品,他们没有得到 FDA 的批准,用于预防、治疗或治愈任何医疗状况、疾病或疾病。我们必须强调,将这些产品以任何形式引入人类或动物的身体都是法律严格禁止的。遵守这些指南对确保研究和实验的法律和道德标准的符合性至关重要。