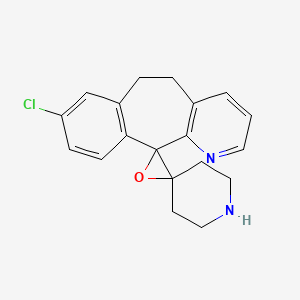
Desloratadine Epoxide
- 点击 快速询问 获取最新报价。
- 提供有竞争力价格的高质量产品,您可以更专注于研究。
描述
Desloratadine Epoxide is a derivative of Desloratadine, a second-generation tricyclic antihistamine. Desloratadine is primarily used to treat allergic conditions such as allergic rhinitis and chronic idiopathic urticaria. This compound is an important metabolite in the biotransformation of Desloratadine, contributing to its pharmacological effects.
准备方法
Synthetic Routes and Reaction Conditions: The synthesis of Desloratadine Epoxide typically involves the epoxidation of Desloratadine. This can be achieved using oxidizing agents such as peracids. A common method is the Prilezhaev epoxidation, where peracids are generated in situ by the oxidation of formic or acetic acids with hydrogen peroxide .
Industrial Production Methods: Industrial production of this compound follows similar synthetic routes but on a larger scale. The process involves careful control of reaction conditions to ensure high yield and purity. The use of continuous flow reactors and advanced purification techniques such as crystallization and chromatography are common in industrial settings.
化学反应分析
Types of Reactions: Desloratadine Epoxide undergoes various chemical reactions, including:
Ring-opening reactions: These can occur under both acidic and basic conditions.
Substitution reactions: Nucleophiles such as water, alcohols, and amines can attack the epoxide ring, leading to the formation of different substituted products.
Common Reagents and Conditions:
Acidic conditions: Hydrochloric acid or sulfuric acid can be used to catalyze the ring-opening of the epoxide.
Basic conditions: Sodium hydroxide or potassium hydroxide can facilitate the nucleophilic attack on the epoxide ring.
Major Products Formed:
Diols: Formed from the hydrolysis of the epoxide ring.
Substituted products: Depending on the nucleophile used, various substituted derivatives can be obtained.
科学研究应用
Desloratadine Epoxide has several applications in scientific research:
Chemistry: It is used as a model compound to study epoxide ring-opening mechanisms and the effects of different reaction conditions.
Biology: Research on this compound helps in understanding the metabolic pathways of Desloratadine and its pharmacokinetics.
Medicine: It contributes to the development of new antihistamine drugs with improved efficacy and reduced side effects.
Industry: this compound is used in the synthesis of other pharmaceutical compounds and as an intermediate in various chemical processes
作用机制
Desloratadine Epoxide exerts its effects by blocking histamine H1 receptors. This prevents histamine from binding to these receptors, thereby reducing allergic symptoms such as nasal congestion, sneezing, and itching. The compound does not readily cross the blood-brain barrier, which minimizes central nervous system side effects such as drowsiness .
相似化合物的比较
Loratadine: The parent compound of Desloratadine, also used as an antihistamine.
Cetirizine: Another second-generation antihistamine with similar uses.
Fexofenadine: A metabolite of Terfenadine, used to treat allergic conditions.
Uniqueness: Desloratadine Epoxide is unique due to its specific metabolic pathway and its role in the pharmacological activity of Desloratadine. Unlike some other antihistamines, it has a lower risk of causing sedation and has a longer duration of action .
生物活性
Desloratadine epoxide, a metabolite of desloratadine, is an important compound in the study of antihistamines. Understanding its biological activity is crucial for evaluating its therapeutic potential and safety profile. This article explores the biological activity of this compound, highlighting its pharmacodynamics, pharmacokinetics, relevant case studies, and research findings.
1. Overview of Desloratadine and Its Metabolites
Desloratadine is a non-sedating antihistamine that primarily acts as a selective H1 receptor antagonist. It is the active metabolite of loratadine and exhibits a higher affinity for H1 receptors compared to its parent compound. This compound is formed through the metabolic oxidation of desloratadine and is believed to contribute to its overall pharmacological effects.
Desloratadine functions by blocking the action of endogenous histamine at H1 receptors located in various tissues, including the respiratory tract and vasculature. This blockade leads to relief from symptoms associated with allergic reactions, such as nasal congestion and watery eyes. The binding affinity of desloratadine to H1 receptors is approximately 15-fold higher than that of loratadine itself .
3. Pharmacokinetics
The pharmacokinetic profile of this compound has not been extensively studied; however, insights can be drawn from desloratadine's pharmacokinetics:
- Absorption : Desloratadine is well absorbed after oral administration, with maximum plasma concentrations achieved within 3 hours.
- Half-life : The terminal half-life ranges from 27 to 120 hours depending on metabolic phenotype .
- Metabolism : Desloratadine undergoes hepatic metabolism primarily via cytochrome P450 enzymes, leading to the formation of several metabolites, including this compound .
4.1 Antihistaminic Activity
This compound retains antihistaminic properties similar to those of desloratadine. In vitro studies have demonstrated that it can effectively inhibit histamine-induced responses in various tissues .
4.2 Safety Profile
Clinical trials have indicated that desloratadine has a favorable safety profile, with minimal adverse effects reported. Notably, it does not cause sedation or significant cardiovascular effects at therapeutic doses . However, a recent study highlighted an increased incidence of seizures among individuals under 20 years during periods of desloratadine exposure, indicating a need for caution in this demographic .
5.1 Seizure Incidence Study
A post-authorization safety study conducted in Nordic countries assessed the association between desloratadine exposure and seizure incidence. The study included over 1.8 million first-time users and found a 46% increased incidence rate of seizures during exposed periods compared to unexposed periods, particularly in children aged 0-5 years .
Age Group | Incidence Rate (per 100,000 person-years) | Adjusted Incidence Rate Ratio (aIRR) |
---|---|---|
Children (0-5) | 31.6 | 1.85 |
Adults (20+) | 21.7 | 1.01 |
5.2 Pharmacogenetics
Research indicates variability in desloratadine metabolism due to genetic polymorphisms affecting cytochrome P450 enzymes. Slow metabolizers exhibit significantly higher plasma concentrations of desloratadine and its metabolites, which may influence both efficacy and safety profiles .
6. Conclusion
This compound represents an important aspect of the pharmacological activity associated with desloratadine. Its role as an H1 receptor antagonist contributes to the therapeutic effects observed in allergic conditions while maintaining a favorable safety profile in most populations. However, emerging data regarding seizure risk in younger populations necessitates further investigation into the long-term effects and safety considerations for this compound.
常见问题
Basic Research Questions
Q. What are the standard analytical methods for quantifying Desloratadine Epoxide in pharmaceutical formulations?
Spectrophotometry (UV-Vis) and high-performance liquid chromatography (HPLC) are widely used. For UV-Vis, ensure absorbance measurements at validated wavelengths (e.g., 232 nm for desloratadine) using calibrated spectrophotometers . HPLC methods require optimization of mobile phases (e.g., ethyl acetate/n-butanol/ammonia/methanol mixtures) and column selection (e.g., C18 reversed-phase) to separate this compound from matrix components. Validate linearity, precision, and accuracy per ICH Q2(R1) guidelines .
Q. How should researchers validate an analytical procedure for this compound to ensure reliability?
Follow ICH validation parameters:
- Linearity : Generate a calibration curve (e.g., 0.05–3.00 µg/spot for TLC) with R² ≥ 0.995 .
- Precision : Perform intra-day and inter-day assays (n=6) with ≤2% RSD for repeatability .
- Accuracy : Use spike-and-recovery tests (90–110% recovery) .
- LOD/LOQ : Calculate via signal-to-noise ratios (3:1 for LOD, 10:1 for LOQ) .
Q. What are the key challenges in sample preparation for this compound assays, and how can they be mitigated?
Manual tablet grinding introduces inhomogeneity, leading to assay variability. Mitigate by:
- Using mechanical homogenization (e.g., ball milling).
- Implementing particle size analysis (e.g., sieving to ≤100 µm).
- Validating homogenization with acceptance criteria for relative standard deviation (RSD ≤2%) .
Advanced Research Questions
Q. What experimental design considerations are critical for successful transfer of this compound assay methods between laboratories?
- Pre-transfer phase : Reproduce the method in the receiving laboratory (RU) using reference standards and identical equipment (e.g., Mettler Toledo balances, Lambda 25 spectrophotometers) .
- Acceptance criteria : Define based on target measurement uncertainty (e.g., ±2% for accuracy, ≤1.5% RSD for precision) .
- Statistical analysis : Use ANOVA to compare inter-lab variability and confirm equivalence (p > 0.05) .
Q. How can researchers resolve inconsistencies in this compound assay data caused by sample inhomogeneity?
- Root-cause analysis : Conduct particle size distribution tests and homogeneity validation via stratified sampling .
- Corrective actions : Implement automated grinding protocols or sonication-assisted dissolution to improve uniformity .
- Data normalization : Apply correction factors based on batch-specific inhomogeneity metrics .
Q. What methodologies are recommended for assessing the stability of this compound under accelerated degradation conditions?
- Stress testing : Expose samples to UV light (254 nm), heat (40–80°C), and acidic/alkaline hydrolysis.
- Analytical monitoring : Use GC-MS to identify degradation products (e.g., epoxide ring-opening derivatives) and quantify remaining this compound .
- Kinetic modeling : Calculate degradation rate constants (k) and shelf-life predictions using Arrhenius equations .
Q. How can metrological approaches be integrated with pharmacopeial guidelines to enhance the accuracy of this compound assays?
Combine the State Pharmacopeia of Ukraine (SPhU) metrological framework (e.g., uncertainty budgeting) with USP Chapter 〈111〉 design-of-experiment principles. For example:
- Quantify uncertainty contributions (e.g., balance calibration, volumetric errors) .
- Validate methods against USP acceptance thresholds for bias (±1.5%) and precision (RSD ≤2%) .
Q. What strategies are effective in minimizing measurement uncertainty during this compound quantification?
- Uncertainty budgeting : Calculate combined standard uncertainty (uc) from individual sources (e.g., pipetting, instrument drift) .
- Robust calibration : Use certified reference materials (CRMs) and traceable standards .
- Interlaboratory comparisons : Participate in proficiency testing programs to validate uncertainty estimates .
Q. How should chromatographic methods (e.g., HPLC, GC-MS) be optimized for detecting this compound and its metabolites?
- Column selection : Use polar-embedded columns (e.g., Zorbax SB-Phenyl) for metabolite separation .
- Mobile phase optimization : Adjust pH (2.5–3.5) and organic modifier ratios (e.g., acetonitrile:buffer 55:45) to enhance resolution .
- Detection parameters : Set MS ionization to positive mode (ESI+) with m/z 311 for this compound .
Q. What advanced statistical tools are appropriate for analyzing variability in this compound assay results across multiple batches?
- Multivariate analysis : Apply principal component analysis (PCA) to identify batch-specific variability sources .
- Control charts : Monitor process stability using X-bar and R charts with ±3σ limits .
- Bayesian inference : Model batch-to-batch variability using Markov Chain Monte Carlo (MCMC) simulations .
属性
InChI |
InChI=1S/C19H19ClN2O/c20-15-5-6-16-14(12-15)4-3-13-2-1-9-22-17(13)19(16)18(23-19)7-10-21-11-8-18/h1-2,5-6,9,12,21H,3-4,7-8,10-11H2 |
Source
|
---|---|---|
Source | PubChem | |
URL | https://pubchem.ncbi.nlm.nih.gov | |
Description | Data deposited in or computed by PubChem | |
InChI Key |
MDTVXCIKQZSGGH-UHFFFAOYSA-N |
Source
|
Source | PubChem | |
URL | https://pubchem.ncbi.nlm.nih.gov | |
Description | Data deposited in or computed by PubChem | |
Canonical SMILES |
C1CC2=C(C=CC(=C2)Cl)C3(C4=C1C=CC=N4)C5(O3)CCNCC5 |
Source
|
Source | PubChem | |
URL | https://pubchem.ncbi.nlm.nih.gov | |
Description | Data deposited in or computed by PubChem | |
Molecular Formula |
C19H19ClN2O |
Source
|
Source | PubChem | |
URL | https://pubchem.ncbi.nlm.nih.gov | |
Description | Data deposited in or computed by PubChem | |
Molecular Weight |
326.8 g/mol |
Source
|
Source | PubChem | |
URL | https://pubchem.ncbi.nlm.nih.gov | |
Description | Data deposited in or computed by PubChem | |
体外研究产品的免责声明和信息
请注意,BenchChem 上展示的所有文章和产品信息仅供信息参考。 BenchChem 上可购买的产品专为体外研究设计,这些研究在生物体外进行。体外研究,源自拉丁语 "in glass",涉及在受控实验室环境中使用细胞或组织进行的实验。重要的是要注意,这些产品没有被归类为药物或药品,他们没有得到 FDA 的批准,用于预防、治疗或治愈任何医疗状况、疾病或疾病。我们必须强调,将这些产品以任何形式引入人类或动物的身体都是法律严格禁止的。遵守这些指南对确保研究和实验的法律和道德标准的符合性至关重要。