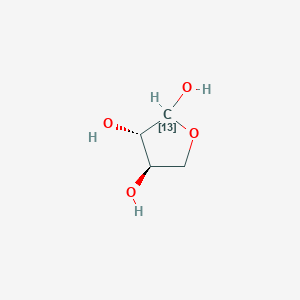
D-苏糖-13C
- 点击 快速询问 获取最新报价。
- 提供有竞争力价格的高质量产品,您可以更专注于研究。
描述
(3S,4R)-(213C)Oxolane-2,3,4-triol, also known as (3S,4R)-(213C)Oxolane-2,3,4-triol, is a useful research compound. Its molecular formula is C4H8O4 and its molecular weight is 121.096. The purity is usually 95%.
BenchChem offers high-quality (3S,4R)-(213C)Oxolane-2,3,4-triol suitable for many research applications. Different packaging options are available to accommodate customers' requirements. Please inquire for more information about (3S,4R)-(213C)Oxolane-2,3,4-triol including the price, delivery time, and more detailed information at info@benchchem.com.
科学研究应用
生物分子核磁共振研究
D-苏糖-13C 可用于生物分子核磁共振 (NMR) 研究 . NMR 是研究分子结构和动力学的强大工具,使用 13C 标记化合物可以提高 NMR 实验的灵敏度和分辨率。
代谢研究
this compound 可用于代谢研究 . 通过跟踪 13C 标签在代谢产物中的掺入,研究人员可以深入了解代谢途径和通量。
代谢组学中的化合物鉴定
在代谢组学应用中,已描述了使用 13C(富集或自然丰度) . 一种称为同位素比率异常分析 (IROA) 的技术利用用 5%(测试)和 95%(对照)13C 同位素标记的样品 . 这种标记策略导致了特征性的同位素模式,可以区分生物信号和伪影,并得出确切的碳原子数,从而显著减少可能的分子式 .
天然产物研究
与代谢组学类似,天然产物研究与使用 13C 的目标有很多共同之处 . 这两个领域都最终目标是确定导致特定活性或表型的某种小分子 .
药物机制研究
代谢组学同位素示踪可以提供用于了解药物机制的有用通量信息 . 为此,NMR 具有提供位置同位素富集信息的独特优势 .
戊糖研究
作用机制
Target of Action
D-Threose-13C, also known as (3S,4R)-(213C)Oxolane-2,3,4-triol, is a compound that has been studied for its potential medical and biochemical applications . .
Mode of Action
It is believed to act on multiple pathways, including the regulation of glucose metabolism and the immune system . Additionally, D-Threose-13C is thought to act on mitochondria and the endoplasmic reticulum, and regulate the expression of genes involved in glucose metabolism .
Biochemical Pathways
D-Threose-13C is involved in various biochemical pathways. It has been shown to increase glucose uptake in cells and improve glucose tolerance in diabetic mice . Furthermore, D-Threose-13C has been found to reduce inflammation and improve cardiovascular health . It has also been found to reduce oxidative stress and improve mitochondrial function .
Result of Action
D-Threose-13C has been found to have multiple biochemical and physiological effects. It has been shown to increase glucose uptake in cells, improve glucose tolerance in diabetic mice, reduce inflammation, improve cardiovascular health, reduce oxidative stress, and improve mitochondrial function .
生化分析
Biochemical Properties
D-Threose-13C: plays a significant role in biochemical reactions, particularly in the study of carbohydrate metabolism. It interacts with various enzymes, proteins, and other biomolecules. For instance, it is involved in the synthesis of glucose and mannose derivatives, which are crucial for energy production and storage in cells . The interactions of D-Threose-13C with enzymes such as aldolases and isomerases facilitate the conversion of this compound into other essential sugars, thereby influencing metabolic flux and metabolite levels.
Cellular Effects
The effects of D-Threose-13C on various types of cells and cellular processes are profound. This compound influences cell function by modulating cell signaling pathways, gene expression, and cellular metabolism. For example, D-Threose-13C has been shown to affect the carbonyl migration and epimerization processes in sugars, which are critical for maintaining cellular homeostasis . Additionally, it impacts the biosynthesis of nucleic acids and proteins, thereby affecting overall cellular function and health.
Molecular Mechanism
At the molecular level, D-Threose-13C exerts its effects through specific binding interactions with biomolecules. It can act as a substrate for various enzymes, leading to enzyme activation or inhibition. For instance, the binding of D-Threose-13C to aldolases results in the cleavage and formation of carbon-carbon bonds, which are essential for the synthesis of complex carbohydrates . Furthermore, D-Threose-13C can influence gene expression by modulating the activity of transcription factors and other regulatory proteins.
Temporal Effects in Laboratory Settings
In laboratory settings, the effects of D-Threose-13C change over time. The stability and degradation of this compound are critical factors that influence its long-term effects on cellular function. Studies have shown that D-Threose-13C remains stable under specific conditions, allowing for prolonged observation of its effects in in vitro and in vivo studies . Its degradation can lead to the formation of by-products that may have different biochemical properties and effects on cells.
Dosage Effects in Animal Models
The effects of D-Threose-13C vary with different dosages in animal models. At low doses, this compound can enhance metabolic activity and improve cellular function. At high doses, it may exhibit toxic or adverse effects, such as disrupting cellular homeostasis and causing oxidative stress . Threshold effects have been observed, indicating that there is an optimal dosage range for achieving the desired biochemical outcomes without causing harm to the organism.
Metabolic Pathways
D-Threose-13C: is involved in several metabolic pathways, including glycolysis and the pentose phosphate pathway. It interacts with enzymes such as hexokinases and transketolases, which are essential for the conversion of glucose and other sugars into energy and biosynthetic precursors. The presence of D-Threose-13C in these pathways allows researchers to study the dynamics of metabolic flux and the regulation of metabolite levels in cells.
Transport and Distribution
Within cells and tissues, D-Threose-13C is transported and distributed through specific transporters and binding proteins. These transporters facilitate the uptake and localization of D-Threose-13C in various cellular compartments, ensuring its availability for metabolic processes . The distribution of D-Threose-13C can also affect its accumulation in specific tissues, influencing its overall biochemical effects.
Subcellular Localization
The subcellular localization of D-Threose-13C is crucial for its activity and function. This compound is directed to specific compartments or organelles through targeting signals and post-translational modifications. For example, D-Threose-13C may be localized to the cytoplasm or mitochondria, where it participates in energy production and other metabolic processes . The precise localization of D-Threose-13C ensures its effective involvement in cellular functions and biochemical reactions.
生物活性
(3S,4R)-(213C)Oxolane-2,3,4-triol, also known as D-Threose-13C, is a carbohydrate derivative that has garnered attention for its potential biological activities. This compound is particularly significant in the context of metabolic studies and therapeutic applications due to its involvement in various biochemical pathways.
D-Threose-13C is a labeled form of the tetrose saccharide D-threose, where the carbon at position 4 is enriched with the carbon-13 isotope. Its molecular formula is C4H8O4, and it features hydroxyl groups at positions 2, 3, and 4 of the oxolane ring. This unique structure facilitates its interaction with biological molecules.
The biological activity of D-Threose-13C is primarily attributed to its role in glucose metabolism and immune regulation. It has been shown to:
- Increase Glucose Uptake : D-Threose-13C enhances glucose uptake in various cell types, which is particularly beneficial in diabetic models.
- Improve Glucose Tolerance : In studies involving diabetic mice, administration of D-Threose-13C led to improved glucose tolerance, suggesting its potential as a therapeutic agent for managing diabetes.
- Reduce Inflammation : The compound has demonstrated anti-inflammatory properties, contributing to cardiovascular health by mitigating oxidative stress and enhancing mitochondrial function.
Biochemical Pathways
D-Threose-13C participates in several critical biochemical pathways:
- Glycolysis : It acts as a substrate for enzymes involved in glycolytic pathways, facilitating energy production.
- Pentose Phosphate Pathway : The compound's involvement in this pathway aids in nucleotide synthesis and cellular redox balance.
Research Findings and Case Studies
Several studies have explored the effects of D-Threose-13C on biological systems:
-
In Vitro Studies :
- In laboratory settings, D-Threose-13C has been shown to remain stable under physiological conditions, allowing for extended observation of its effects on cellular functions.
- It has been utilized in NMR spectroscopy studies due to its carbon-13 labeling, providing insights into protein dynamics at an atomic level.
-
Animal Models :
- A study on diabetic mice indicated that D-Threose-13C significantly improved glucose metabolism and reduced markers of inflammation compared to control groups.
- Clinical Implications :
Comparative Analysis
To better understand the biological activity of D-Threose-13C, a comparison with other similar compounds can be beneficial.
Compound Name | Structure Type | Key Biological Activity |
---|---|---|
D-Threose | Tetrose | Glucose metabolism enhancement |
D-Erythrose | Tetrose | Nucleotide synthesis |
(2R)-Oxolane-2,3,4-triol | Triol | Used in NMR studies; metabolic pathways |
属性
IUPAC Name |
(3S,4R)-(213C)oxolane-2,3,4-triol |
Source
|
---|---|---|
Source | PubChem | |
URL | https://pubchem.ncbi.nlm.nih.gov | |
Description | Data deposited in or computed by PubChem | |
InChI |
InChI=1S/C4H8O4/c5-2-1-8-4(7)3(2)6/h2-7H,1H2/t2-,3+,4?/m1/s1/i4+1 |
Source
|
Source | PubChem | |
URL | https://pubchem.ncbi.nlm.nih.gov | |
Description | Data deposited in or computed by PubChem | |
InChI Key |
FMAORJIQYMIRHF-GHNFDQGXSA-N |
Source
|
Source | PubChem | |
URL | https://pubchem.ncbi.nlm.nih.gov | |
Description | Data deposited in or computed by PubChem | |
Canonical SMILES |
C1C(C(C(O1)O)O)O |
Source
|
Source | PubChem | |
URL | https://pubchem.ncbi.nlm.nih.gov | |
Description | Data deposited in or computed by PubChem | |
Isomeric SMILES |
C1[C@H]([C@@H]([13CH](O1)O)O)O |
Source
|
Source | PubChem | |
URL | https://pubchem.ncbi.nlm.nih.gov | |
Description | Data deposited in or computed by PubChem | |
Molecular Formula |
C4H8O4 |
Source
|
Source | PubChem | |
URL | https://pubchem.ncbi.nlm.nih.gov | |
Description | Data deposited in or computed by PubChem | |
Molecular Weight |
121.10 g/mol |
Source
|
Source | PubChem | |
URL | https://pubchem.ncbi.nlm.nih.gov | |
Description | Data deposited in or computed by PubChem | |
体外研究产品的免责声明和信息
请注意,BenchChem 上展示的所有文章和产品信息仅供信息参考。 BenchChem 上可购买的产品专为体外研究设计,这些研究在生物体外进行。体外研究,源自拉丁语 "in glass",涉及在受控实验室环境中使用细胞或组织进行的实验。重要的是要注意,这些产品没有被归类为药物或药品,他们没有得到 FDA 的批准,用于预防、治疗或治愈任何医疗状况、疾病或疾病。我们必须强调,将这些产品以任何形式引入人类或动物的身体都是法律严格禁止的。遵守这些指南对确保研究和实验的法律和道德标准的符合性至关重要。