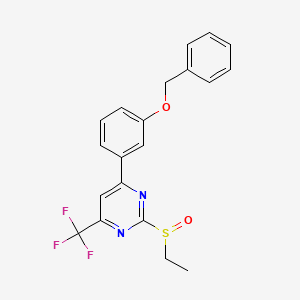
Betp
作用机制
BETP 通过与胰高血糖素样肽-1 受体(一种 G 蛋白偶联受体)结合发挥作用。结合后,this compound 作为一种正向变构调节剂,增强受体对其天然配体胰高血糖素样肽-1 的反应。这导致胰岛素分泌增加和葡萄糖代谢改善。 涉及的分子靶标包括受体本身和下游信号通路,如环腺苷酸途径 .
生化分析
Biochemical Properties
BetP transports betaine with high specificity, which under hyperosmotic conditions is accumulated in molar amounts against the concentration gradient into the cytoplasm . The transporter exploits the inward directed sodium gradient across the membrane to energize betaine transport in a stoichiometry of 2 Na+:1 betaine . It is assumed that this compound senses changes of the physical state of the membrane via its C-terminal domain .
Cellular Effects
This compound has a significant impact on cellular processes, particularly in response to osmotic stress. It senses an osmotic upshift via its osmosensory C-terminal domain and responds rapidly with a steep increase in transport rate . This rapid response helps the cell to adapt to changes in the external environment and maintain homeostasis.
Molecular Mechanism
The molecular mechanism of this compound involves a complex interplay of structural changes and interactions with other molecules. X-ray crystallography has identified four putative K+ interaction sites at the C-terminal domains of this compound . The presence of K+ results in asymmetric partial unfolding of the C-terminal domains, which is an intermediate between the downregulated state and the conformation observed in crystal structures .
Temporal Effects in Laboratory Settings
In laboratory settings, the effects of this compound can change over time. For instance, the activation of this compound is highly cooperative, with K+ binding to this compound in vivo showing a high degree of cooperativity . This suggests that the activity of this compound can be modulated by changes in the intracellular environment.
Dosage Effects in Animal Models
While specific dosage effects of this compound in animal models have not been extensively studied, it is known that the activity of this compound is regulated by the intracellular concentration of K+ . This suggests that the effects of this compound could potentially vary with different dosages of K+.
Metabolic Pathways
This compound is involved in the transport of betaine, a process that is crucial for the osmotic stress response in bacteria . This process is part of a larger metabolic pathway that helps the cell to adapt to changes in the external environment.
Transport and Distribution
This compound is a membrane transporter, and it is distributed within the cell membrane . It is thought to sense changes in the physical state of the membrane via its C-terminal domain , which may affect its distribution within the membrane.
Subcellular Localization
This compound is localized in the cell membrane, where it functions as a transporter . The C-terminal domain of this compound, which is thought to be involved in sensing osmotic stress, is attached at the membrane surface when this compound is downregulated in the absence of stress .
准备方法
合成路线和反应条件
BETP 可以通过多步合成,包括形成嘧啶核心,然后引入苄氧基苯基、乙基亚磺酰基和三氟甲基。合成通常包括:
嘧啶核心的形成: 可以通过在促进环化的条件下使适当的前体发生反应来实现。
苄氧基苯基的引入: 该步骤涉及使用钯催化的交叉偶联反应将苄氧基苯基衍生物与嘧啶核心偶联。
乙基亚磺酰基的引入: 可以通过使用过氧化氢等氧化剂将乙硫基氧化为乙基亚磺酰基来完成。
三氟甲基的引入: 该步骤涉及使用三氟甲基碘等试剂将三氟甲基添加到嘧啶核心.
工业生产方法
This compound 的工业生产遵循类似的合成路线,但针对大规模生产进行了优化。这包括使用高通量筛选和优化反应条件,以最大限度地提高产率和纯度。 该过程还可以包括使用自动化合成设备和连续流动反应器来提高效率和可扩展性 .
化学反应分析
反应类型
BETP 经历各种化学反应,包括:
氧化: 乙硫基可以氧化为乙基亚磺酰基。
还原: 乙基亚磺酰基可以还原回乙硫基。
取代: 三氟甲基可以在适当条件下被其他官能团取代.
常用试剂和条件
氧化: 过氧化氢通常用作氧化剂。
还原: 可以使用硼氢化钠等还原剂。
主要产品
从这些反应中形成的主要产物包括具有改性官能团的 this compound 衍生物,例如乙硫基、乙基亚磺酰基和取代的三氟甲基 .
科学研究应用
BETP 具有多种科学研究应用,包括:
化学: this compound 用作研究胰高血糖素样肽-1 受体及其在葡萄糖代谢中的作用的工具化合物。
生物学: this compound 用于研究胰岛素分泌和葡萄糖稳态中涉及的信号通路。
医学: this compound 正在探索作为治疗 2 型糖尿病和肥胖症的潜在治疗剂。
相似化合物的比较
BETP 在胰高血糖素样肽-1 受体激动剂中是独一无二的,因为它具有小分子性质和口服生物利用度。类似的化合物包括:
利拉鲁肽: 胰高血糖素样肽-1 受体的肽激动剂,通过注射给药。
司美格鲁肽: 另一种肽激动剂,也通过注射给药。
属性
IUPAC Name |
2-ethylsulfinyl-4-(3-phenylmethoxyphenyl)-6-(trifluoromethyl)pyrimidine | |
---|---|---|
Source | PubChem | |
URL | https://pubchem.ncbi.nlm.nih.gov | |
Description | Data deposited in or computed by PubChem | |
InChI |
InChI=1S/C20H17F3N2O2S/c1-2-28(26)19-24-17(12-18(25-19)20(21,22)23)15-9-6-10-16(11-15)27-13-14-7-4-3-5-8-14/h3-12H,2,13H2,1H3 | |
Source | PubChem | |
URL | https://pubchem.ncbi.nlm.nih.gov | |
Description | Data deposited in or computed by PubChem | |
InChI Key |
NTDFYGSSDDMNHI-UHFFFAOYSA-N | |
Source | PubChem | |
URL | https://pubchem.ncbi.nlm.nih.gov | |
Description | Data deposited in or computed by PubChem | |
Canonical SMILES |
CCS(=O)C1=NC(=CC(=N1)C(F)(F)F)C2=CC(=CC=C2)OCC3=CC=CC=C3 | |
Source | PubChem | |
URL | https://pubchem.ncbi.nlm.nih.gov | |
Description | Data deposited in or computed by PubChem | |
Molecular Formula |
C20H17F3N2O2S | |
Source | PubChem | |
URL | https://pubchem.ncbi.nlm.nih.gov | |
Description | Data deposited in or computed by PubChem | |
Molecular Weight |
406.4 g/mol | |
Source | PubChem | |
URL | https://pubchem.ncbi.nlm.nih.gov | |
Description | Data deposited in or computed by PubChem | |
CAS No. |
1371569-69-5 | |
Record name | 1371569-69-5 | |
Source | European Chemicals Agency (ECHA) | |
URL | https://echa.europa.eu/information-on-chemicals | |
Description | The European Chemicals Agency (ECHA) is an agency of the European Union which is the driving force among regulatory authorities in implementing the EU's groundbreaking chemicals legislation for the benefit of human health and the environment as well as for innovation and competitiveness. | |
Explanation | Use of the information, documents and data from the ECHA website is subject to the terms and conditions of this Legal Notice, and subject to other binding limitations provided for under applicable law, the information, documents and data made available on the ECHA website may be reproduced, distributed and/or used, totally or in part, for non-commercial purposes provided that ECHA is acknowledged as the source: "Source: European Chemicals Agency, http://echa.europa.eu/". Such acknowledgement must be included in each copy of the material. ECHA permits and encourages organisations and individuals to create links to the ECHA website under the following cumulative conditions: Links can only be made to webpages that provide a link to the Legal Notice page. | |
Q1: What is the primary function of BetP?
A1: this compound is a secondary active transporter that facilitates the uptake of the compatible solute glycine betaine into the cytoplasm of Corynebacterium glutamicum [, ]. This process is driven by the co-transport of sodium ions [, ].
Q2: What is the physiological role of glycine betaine accumulation in C. glutamicum?
A2: Glycine betaine acts as a compatible solute, protecting the cell from the detrimental effects of hyperosmotic stress [, ]. It helps maintain cellular turgor pressure and enzyme function under high osmolarity conditions [, , ].
Q3: How does this compound contribute to the osmotic stress response of C. glutamicum?
A3: this compound acts as both an osmosensor and an osmoregulator [, , , ]. It senses hyperosmotic conditions and responds by increasing its transport rate to rapidly accumulate glycine betaine [, ].
Q4: Does C. glutamicum rely solely on this compound for compatible solute uptake?
A4: No, C. glutamicum possesses four secondary carriers for compatible solutes: this compound, EctP, LcoP, and ProP, each with distinct substrate specificities and affinities [, ].
Q5: What is the oligomeric state of this compound?
A5: this compound functions as a homotrimer, meaning it is composed of three identical protein subunits [, , ].
Q6: Which domains of this compound are involved in sensing osmotic stress?
A6: Both the N-terminal and C-terminal cytoplasmic extensions of this compound contribute to osmosensing and regulation [, ].
Q7: What is the role of the C-terminal domain in this compound regulation?
A7: The C-terminal domain acts as a primary osmosensor, directly sensing changes in the cytoplasmic potassium ion (K+) concentration as an indicator of hyperosmotic stress [, , ].
Q8: How does the C-terminal domain transduce the osmotic signal?
A8: Upon K+ binding, the C-terminal domain undergoes conformational changes, leading to a rearrangement of the this compound trimer and activation of the transporter [, ]. This involves switching interactions between the C-terminal domain, the N-terminal domain, and the membrane lipids [, ].
Q9: What is the role of the N-terminal domain in this compound regulation?
A9: The N-terminal domain contains negatively charged clusters that interact with the positively charged C-terminal domain, modulating the activation state of this compound []. This interaction is crucial for the osmoregulatory response.
Q10: What is the significance of the five transmembrane-helix inverted-topology repeat (FIRL) fold in this compound?
A10: this compound, like other sodium-coupled transporters, adopts the FIRL fold, which is believed to be essential for the alternating access mechanism that allows for substrate transport across the membrane [].
Q11: What are the primary stimuli that activate this compound?
A11: this compound is activated by: * Increased internal K+ concentration: This is the primary signal for hyperosmotic stress response [, ].* Changes in the physical state of the membrane: The exact nature of this signal is not fully elucidated, but it is thought to involve alterations in membrane fluidity or lipid composition [, , ].
Q12: How does K+ activate this compound?
A12: K+ binds to specific sites within the C-terminal domain of this compound []. This binding is highly cooperative and triggers conformational changes that ultimately lead to transporter activation [, ].
Q13: How do changes in membrane composition affect this compound activity?
A13: The presence of negatively charged lipids, particularly phosphatidylglycerol, is crucial for this compound activity and osmoregulation [, ]. The C-terminal domain interacts with these lipids, and these interactions are modulated by K+ binding [].
Q14: Is this compound subject to regulation after initial activation?
A14: Yes, during the adaptation phase to hyperosmotic stress, this compound activity decreases despite the continued presence of a high internal K+ concentration []. This adaptation regulation is independent of K+ and might be related to changes in the hydration status of this compound or altered protein-membrane interactions [].
Q15: Does this compound exhibit any transport activity in the absence of osmotic stress?
A15: Yes, this compound displays a basal level of activity even under low osmolarity conditions. This ensures a minimal uptake of compatible solutes and allows for a rapid response upon osmotic upshift [].
Q16: What structural techniques have been employed to study this compound?
A16: Several techniques have been used to elucidate this compound structure, including:* X-ray crystallography: Provided high-resolution structures of this compound in various conformations [, ].* Cryo-electron microscopy (CryoEM): Revealed the structure of this compound in a lipid bilayer environment [].* Electron paramagnetic resonance (EPR) spectroscopy: Provided insights into the dynamics of this compound in response to different stimuli [, ].* Single-molecule FRET: Offered information about conformational changes and heterogeneity of this compound [].
Q17: What insights have structural studies provided into the mechanism of this compound activation?
A17: Structural studies, combined with biochemical and biophysical data, suggest that this compound undergoes a series of conformational changes during activation [, ]. * In the absence of K+, the C-terminal domains are located near the membrane surface, representing a downregulated state []. * Upon K+ binding, the C-terminal domains partially unfold and reorient, leading to a rearrangement of the transporter and facilitating substrate binding and transport [, ].
Q18: How does this compound compare to other osmoregulated transporters?
A18: While this compound's specific K+-dependent regulation is unique, the concept of sensing domain unfolding/refolding as a mechanism for responding to hyperosmotic stress is likely shared by other osmoregulated transporters [].
Q19: Are there any biotechnological applications of this compound or related transporters?
A19: Understanding the mechanisms of osmoregulation in bacteria like C. glutamicum has implications for optimizing their use in biotechnology [, ]. Engineering strains with enhanced osmotolerance could improve their performance in industrial processes.
体外研究产品的免责声明和信息
请注意,BenchChem 上展示的所有文章和产品信息仅供信息参考。 BenchChem 上可购买的产品专为体外研究设计,这些研究在生物体外进行。体外研究,源自拉丁语 "in glass",涉及在受控实验室环境中使用细胞或组织进行的实验。重要的是要注意,这些产品没有被归类为药物或药品,他们没有得到 FDA 的批准,用于预防、治疗或治愈任何医疗状况、疾病或疾病。我们必须强调,将这些产品以任何形式引入人类或动物的身体都是法律严格禁止的。遵守这些指南对确保研究和实验的法律和道德标准的符合性至关重要。