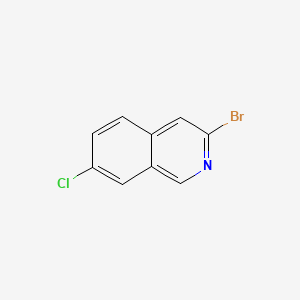
3-溴-7-氯异喹啉
- 点击 快速询问 获取最新报价。
- 提供有竞争力价格的高质量产品,您可以更专注于研究。
描述
3-Bromo-7-chloroisoquinoline is a chemical compound with the molecular formula C9H5BrClN and a molecular weight of 242.5 . It is a solid powder at room temperature .
Molecular Structure Analysis
The InChI code for 3-Bromo-7-chloroisoquinoline is1S/C9H5BrClN/c10-9-4-6-1-2-8(11)3-7(6)5-12-9/h1-5H
. This indicates that the compound has a benzene ring fused with a pyridine moiety, with bromine and chlorine substituents . Physical And Chemical Properties Analysis
3-Bromo-7-chloroisoquinoline is a solid powder at room temperature . It has a molecular weight of 242.5 . The compound’s InChI code is1S/C9H5BrClN/c10-9-4-6-1-2-8(11)3-7(6)5-12-9/h1-5H
.
科学研究应用
反应和合成
- 3-溴-7-氯异喹啉及类似化合物在各种合成途径和反应中得到探讨。例如,一项研究讨论了涉及4-卤代异喹啉和氨中酰胺离子的反应,在特定条件下显示了3-氯异喹啉的显著反应(Zoltewicz & Oestreich, 1991)。另一项研究重点介绍了抗肿瘤生物碱卢诺汀A的氟甲基含量类似物的合成,其中包括3-溴甲基-2-氯-4-氟甲基喹啉(Golubev et al., 2010)。
化学合成和表征
- 各种研究集中在喹啉衍生物的合成和表征上。例如,关于合成2-(4-((6-溴-3-硝基喹啉-4-基)氨基)苯基)-2-甲基丙腈的研究展示了溴喹啉化合物在PI3K/mTOR抑制剂合成中的潜力(Lei et al., 2015)。另一项研究报告了吡啶盐酸盐用于从喹啉系列的溴衍生物合成氯化合物(Mongin et al., 1996)。
药物化学和药理学
- 3-溴-7-氯异喹啉结构被用于开发各种药理活性化合物。一个显著的例子是合成N-(2-氨基乙基)-5-氯异喹啉-8-磺酰胺,一种选择性酪蛋白激酶I抑制剂,为该酶的生物学意义提供了见解(Chijiwa et al., 1989)。
光谱和结构研究
- 对7-溴-5-氯-8-羟基喹啉等化合物进行振动光谱研究和从头算和密度泛函理论研究,提供了有关它们结构和振动性质的宝贵数据,增进了对这类化合物的理解(Arjunan et al., 2009)。
安全和危害
3-Bromo-7-chloroisoquinoline is classified under GHS07. It may cause skin irritation (H315), serious eye irritation (H319), and respiratory irritation (H335) . Precautionary measures include avoiding breathing dust and contact with skin and eyes, using personal protective equipment, and ensuring adequate ventilation .
作用机制
Target of Action
It is a synthetic fine chemical useful for studying the biochemistry of macromolecules .
Mode of Action
It’s known that the compound is a nitrogen heterocycle, and it’s reactive with bromine and chlorine
Result of Action
As a synthetic fine chemical, it’s primarily used for studying the biochemistry of macromolecules , suggesting it may have broad applications in biochemical research.
Action Environment
The action, efficacy, and stability of 3-Bromo-7-chloroisoquinoline can be influenced by environmental factors. For instance, it’s recommended to store the compound at 2-8°C under dry conditions . This suggests that temperature and humidity could affect its stability and, potentially, its efficacy.
属性
IUPAC Name |
3-bromo-7-chloroisoquinoline |
Source
|
---|---|---|
Source | PubChem | |
URL | https://pubchem.ncbi.nlm.nih.gov | |
Description | Data deposited in or computed by PubChem | |
InChI |
InChI=1S/C9H5BrClN/c10-9-4-6-1-2-8(11)3-7(6)5-12-9/h1-5H |
Source
|
Source | PubChem | |
URL | https://pubchem.ncbi.nlm.nih.gov | |
Description | Data deposited in or computed by PubChem | |
InChI Key |
ZLAFRLYZTMTUOQ-UHFFFAOYSA-N |
Source
|
Source | PubChem | |
URL | https://pubchem.ncbi.nlm.nih.gov | |
Description | Data deposited in or computed by PubChem | |
Canonical SMILES |
C1=CC(=CC2=CN=C(C=C21)Br)Cl |
Source
|
Source | PubChem | |
URL | https://pubchem.ncbi.nlm.nih.gov | |
Description | Data deposited in or computed by PubChem | |
Molecular Formula |
C9H5BrClN |
Source
|
Source | PubChem | |
URL | https://pubchem.ncbi.nlm.nih.gov | |
Description | Data deposited in or computed by PubChem | |
DSSTOX Substance ID |
DTXSID90725497 |
Source
|
Record name | 3-Bromo-7-chloroisoquinoline | |
Source | EPA DSSTox | |
URL | https://comptox.epa.gov/dashboard/DTXSID90725497 | |
Description | DSSTox provides a high quality public chemistry resource for supporting improved predictive toxicology. | |
Molecular Weight |
242.50 g/mol |
Source
|
Source | PubChem | |
URL | https://pubchem.ncbi.nlm.nih.gov | |
Description | Data deposited in or computed by PubChem | |
Product Name |
3-Bromo-7-chloroisoquinoline | |
CAS RN |
1246552-90-8 |
Source
|
Record name | 3-Bromo-7-chloroisoquinoline | |
Source | EPA DSSTox | |
URL | https://comptox.epa.gov/dashboard/DTXSID90725497 | |
Description | DSSTox provides a high quality public chemistry resource for supporting improved predictive toxicology. | |
体外研究产品的免责声明和信息
请注意,BenchChem 上展示的所有文章和产品信息仅供信息参考。 BenchChem 上可购买的产品专为体外研究设计,这些研究在生物体外进行。体外研究,源自拉丁语 "in glass",涉及在受控实验室环境中使用细胞或组织进行的实验。重要的是要注意,这些产品没有被归类为药物或药品,他们没有得到 FDA 的批准,用于预防、治疗或治愈任何医疗状况、疾病或疾病。我们必须强调,将这些产品以任何形式引入人类或动物的身体都是法律严格禁止的。遵守这些指南对确保研究和实验的法律和道德标准的符合性至关重要。