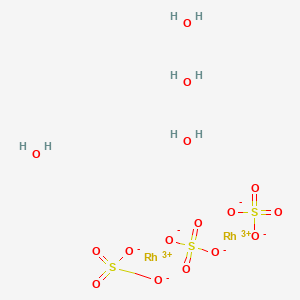
RHODIUM (III) SULFATE
描述
RHODIUM (III) SULFATE is an inorganic compound with the formula Rh₂(SO₄)₃·4H₂O. It is a red crystalline solid that is moderately soluble in water and acids. This compound is notable for its use in various industrial and scientific applications, particularly in the field of catalysis and electroplating .
作用机制
Rhodium(III) sulfate, also known as rhodium(3+);trisulfate;tetrahydrate or Rhodium(III) sulfate tetrahydrate, is an inorganic compound with the formula Rh2(SO4)3 . It is a red crystalline solid that has been studied for its various therapeutic activities .
Target of Action
Rhodium complexes have been found to interact with various biological targets, including DNA, RNA, protein kinases, UPS, KDM5A, and other biomolecules of relevance . These interactions are believed to be responsible for the broad range of therapeutic activities displayed by rhodium complexes .
Mode of Action
The mode of action of Rhodium(III) sulfate involves its interaction with these biological targets. For instance, some rhodium complexes have been found to interact with DNA, leading to inhibition of DNA replication and consequently, death of cancer cells . Rhodium complexes can also interact with RNA .
Biochemical Pathways
Rhodium(III) sulfate is known to affect the cGAS-STING pathway, a key mediator of innate immunity involved in cancer development and treatment . A highly emissive rhodium(III) complex has been reported to bind to mitochondrial DNA (mtDNA), causing the release of mtDNA fragments into the cytoplasm and activating the cGAS-STING pathway .
Result of Action
The interaction of Rhodium(III) sulfate with its biological targets leads to various molecular and cellular effects. For example, the interaction of rhodium complexes with DNA can lead to the death of cancer cells . The activation of the cGAS-STING pathway by a rhodium(III) complex can also lead to immune responses .
Action Environment
The action, efficacy, and stability of Rhodium(III) sulfate can be influenced by various environmental factors. For instance, the compound forms a hydrated solution at high temperatures . It is also used in the production of high-performance coatings by electroplating technique .
准备方法
Synthetic Routes and Reaction Conditions: The first attempts to produce rhodium(III) sulfate were made in 1929 by reacting rhodium(III) hydroxide with sulfuric acid. This reaction initially produced two different types of hydrates: a yellow tetradeca hydrate and a red tetrahydrate . the structural proof for these hydrates was lacking at the time.
Further investigations using X-ray diffraction confirmed the existence of the hexahydrate and tetradecahydrate forms. In 2016, a more efficient production method was reported, which involved heating rhodium metal with sulfuric acid at 400°C to produce the anhydrous compound. Heating at 475°C instead resulted in the formation of the dihydrate .
Industrial Production Methods: Industrial production of rhodium(III) sulfate typically involves the oxidation of rhodium metal using concentrated sulfuric acid. The reaction is carried out under controlled conditions to ensure the desired hydrate form is obtained. The resulting product is then purified and crystallized to achieve high purity levels suitable for various applications .
化学反应分析
Types of Reactions: RHODIUM (III) SULFATE undergoes several types of chemical reactions, including oxidation, reduction, and substitution reactions. These reactions are often facilitated by the presence of common reagents such as sulfuric acid, hydrogen peroxide, and various reducing agents .
Common Reagents and Conditions:
Oxidation: Rhodium(III) sulfate can be oxidized using strong oxidizing agents like hydrogen peroxide.
Reduction: Reduction of rhodium(III) sulfate can be achieved using reducing agents such as sodium borohydride.
Substitution: Substitution reactions often involve the replacement of sulfate ions with other ligands, facilitated by the use of complexing agents.
Major Products: The major products formed from these reactions depend on the specific reagents and conditions used. For example, oxidation reactions may yield higher oxidation state rhodium compounds, while reduction reactions typically produce lower oxidation state rhodium species .
科学研究应用
RHODIUM (III) SULFATE has a wide range of scientific research applications, including:
相似化合物的比较
Rhodium(III) chloride: Another rhodium compound used in catalysis and electroplating.
Rhodium(III) nitrate: Used in similar applications as rhodium(III) sulfate but with different reactivity and solubility properties.
Rhodium(III) acetate: Known for its use in organic synthesis and catalysis.
Uniqueness: RHODIUM (III) SULFATE is unique due to its specific solubility properties and its ability to form stable hydrates. These characteristics make it particularly useful in applications requiring high-purity rhodium sources and controlled reactivity .
生物活性
Rhodium(III) sulfate (Rh₂(SO₄)₃) is an inorganic compound that has garnered attention for its potential biological activities, particularly in the context of cancer treatment. This article explores the biological activity of rhodium(III) sulfate, focusing on its mechanisms of action, cellular effects, and potential therapeutic applications.
Rhodium(III) sulfate is typically encountered as a red crystalline solid. It can be synthesized through various methods, including the reaction of rhodium(III) hydroxide with sulfuric acid or by heating rhodium metal with sulfuric acid at high temperatures . The compound exists in different hydrate forms, with the tetrahydrate and hexahydrate being the most studied.
1. DNA Interaction and Cytotoxicity
Recent studies have shown that rhodium metalloinsertors, which include rhodium(III) sulfate complexes, can bind to DNA mismatches with high affinity. This binding is particularly effective in cells deficient in the mismatch repair (MMR) pathway, which is often exploited in cancer therapy . The following mechanisms have been identified:
- Cell Selectivity : Rhodium complexes exhibit selective cytotoxicity towards MMR-deficient cells. This selectivity is attributed to their ability to localize within the nucleus and bind to DNA mismatches, disrupting cellular processes and inducing cell death .
- Induction of Necrosis : In addition to apoptosis, these complexes can induce necrosis in targeted cancer cells, leading to cell cycle disruption and reduced proliferation rates .
2. Cellular Uptake and Localization
Studies utilizing inductively coupled plasma mass spectrometry (ICP-MS) have demonstrated that rhodium metalloinsertors are rapidly taken up by cells. The compounds localize predominantly in the nucleus, where they exert their cytotoxic effects. Variations in lipophilicity among different metalloinsertors influence their cellular uptake and biological activity .
Case Study 1: Targeting MMR-Deficient Cancer Cells
In a controlled study involving engineered NCI-H23 lung adenocarcinoma cells with suppressed MMR gene expression (MLH1), rhodium metalloinsertors were found to significantly inhibit cell proliferation compared to MMR-proficient controls. This highlights the potential of rhodium complexes as targeted therapies for specific cancer types .
Case Study 2: Comparative Analysis with Other Agents
Comparative studies have shown that traditional DNA-damaging agents like cisplatin are less effective in MMR-deficient cells than rhodium metalloinsertors. This suggests that rhodium compounds may offer a more effective treatment option for patients with tumors exhibiting MMR deficiencies .
Toxicological Considerations
While rhodium(III) sulfate shows promise as a therapeutic agent, it is essential to consider its toxicological profile. Research indicates that exposure to rhodium compounds can lead to reproductive toxicity and alterations in metabolic parameters such as serum lipids and glucose levels . Therefore, understanding the dosage and administration routes is crucial for minimizing adverse effects while maximizing therapeutic benefits.
Summary of Biological Activities
Activity | Description |
---|---|
DNA Binding | High affinity for DNA mismatches, particularly in MMR-deficient cells |
Cell Selectivity | Preferential cytotoxicity towards cancer cells lacking functional MMR |
Induction of Cell Death | Mechanisms include both apoptosis and necrosis |
Cellular Uptake | Rapid uptake with nuclear localization |
Comparative Efficacy | More effective than traditional agents like cisplatin in specific cancer types |
属性
IUPAC Name |
rhodium(3+);trisulfate;tetrahydrate | |
---|---|---|
Source | PubChem | |
URL | https://pubchem.ncbi.nlm.nih.gov | |
Description | Data deposited in or computed by PubChem | |
InChI |
InChI=1S/3H2O4S.4H2O.2Rh/c3*1-5(2,3)4;;;;;;/h3*(H2,1,2,3,4);4*1H2;;/q;;;;;;;2*+3/p-6 | |
Source | PubChem | |
URL | https://pubchem.ncbi.nlm.nih.gov | |
Description | Data deposited in or computed by PubChem | |
InChI Key |
JJITWKYISWZJBI-UHFFFAOYSA-H | |
Source | PubChem | |
URL | https://pubchem.ncbi.nlm.nih.gov | |
Description | Data deposited in or computed by PubChem | |
Canonical SMILES |
O.O.O.O.[O-]S(=O)(=O)[O-].[O-]S(=O)(=O)[O-].[O-]S(=O)(=O)[O-].[Rh+3].[Rh+3] | |
Source | PubChem | |
URL | https://pubchem.ncbi.nlm.nih.gov | |
Description | Data deposited in or computed by PubChem | |
Molecular Formula |
H8O16Rh2S3 | |
Source | PubChem | |
URL | https://pubchem.ncbi.nlm.nih.gov | |
Description | Data deposited in or computed by PubChem | |
DSSTOX Substance ID |
DTXSID401336783 | |
Record name | Rhodium(III) sulfate tetrahydrate | |
Source | EPA DSSTox | |
URL | https://comptox.epa.gov/dashboard/DTXSID401336783 | |
Description | DSSTox provides a high quality public chemistry resource for supporting improved predictive toxicology. | |
Molecular Weight |
566.1 g/mol | |
Source | PubChem | |
URL | https://pubchem.ncbi.nlm.nih.gov | |
Description | Data deposited in or computed by PubChem | |
CAS No. |
15274-78-9 | |
Record name | Rhodium(III) sulfate tetrahydrate | |
Source | EPA DSSTox | |
URL | https://comptox.epa.gov/dashboard/DTXSID401336783 | |
Description | DSSTox provides a high quality public chemistry resource for supporting improved predictive toxicology. | |
体外研究产品的免责声明和信息
请注意,BenchChem 上展示的所有文章和产品信息仅供信息参考。 BenchChem 上可购买的产品专为体外研究设计,这些研究在生物体外进行。体外研究,源自拉丁语 "in glass",涉及在受控实验室环境中使用细胞或组织进行的实验。重要的是要注意,这些产品没有被归类为药物或药品,他们没有得到 FDA 的批准,用于预防、治疗或治愈任何医疗状况、疾病或疾病。我们必须强调,将这些产品以任何形式引入人类或动物的身体都是法律严格禁止的。遵守这些指南对确保研究和实验的法律和道德标准的符合性至关重要。