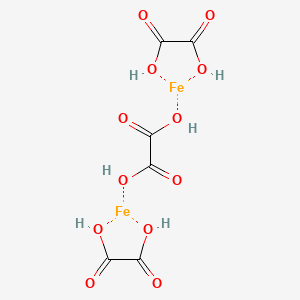
Iron (III) oxalate
描述
Iron (III) oxalate, with the formula Fe₂(C₂O₄)₃·5H₂O, is a coordination compound where iron(III) ions are bridged by oxalate ligands. It exhibits a one-dimensional antiferromagnetic chain structure, characterized by strong intra-chain interactions (exchange coupling constant J = −7.58 cm⁻¹) and negligible inter-chain magnetic coupling . This compound is highly soluble in water, making it a versatile precursor for synthesizing iron-doped materials, such as mesoporous hydroxyapatite (HA) nanoparticles and iron oxides . Its redox activity and photochemical properties also enable applications in photocatalysis and environmental remediation .
准备方法
Oxidation of Iron (II) Oxalate Precursors
Reaction Mechanism and Stoichiometry
The most widely documented method involves oxidizing iron (II) oxalate () to iron (III) oxalate using hydrogen peroxide () in an acidic medium. The process begins with the precipitation of from iron (II) ammonium sulfate () and oxalic acid () :
2\text{C}2\text{O}4 + 2\text{H}2\text{O} \rightarrow \text{FeC}2\text{O}4 \downarrow + 2\text{H}_3\text{O}^+
The precipitate is then oxidized with in the presence of potassium oxalate () to form :
2\text{O}4 + \text{H}2\text{O}2 + 2\text{H}3\text{O}^+ + \text{C}2\text{O}4^{2-} \rightarrow \text{Fe}2(\text{C}2\text{O}4)3 + 4\text{H}2\text{O}
Key parameters include maintaining a reaction temperature of 40°C to prevent premature decomposition of and ensuring a molar ratio of at 2:1:1 .
Procedural Details
-
Precipitation of : Dissolve 1.2030 g of in 3 mL of deionized water acidified with 1–3 drops of 6 M . Add 6 mL of 1 M to precipitate .
-
Oxidation Step: Resuspend the precipitate in 15 mL of saturated , heat to 40°C, and slowly add 30 mL of 3–5% with continuous stirring .
-
Purification: Boil the mixture to decompose excess , then add 12 mL of 1 M to solubilize the product. Ethanol (8 mL, 95%) is added to precipitate , which is filtered and dried .
Yield and Purity
Typical yields range from 60–70%, with impurities primarily arising from incomplete oxidation or residual . Titration with standardized confirms oxalate content (53.2% ± 1.6%), while spectrophotometry at 510 nm quantifies iron (III) at 7.08% ± 0.92% .
Direct Synthesis from Ferric Chloride and Oxalic Acid
Reaction Pathway
This method bypasses the iron (II) intermediate by reacting ferric chloride () directly with oxalic acid. The process involves two stages: hydrolysis of to and subsequent reaction with :
3 + 3\text{KOH} \rightarrow \text{Fe(OH)}3 \downarrow + 3\text{KCl}
3 + 3\text{H}2\text{C}2\text{O}4 \rightarrow \text{Fe}2(\text{C}2\text{O}4)3 + 6\text{H}_2\text{O}
Experimental Protocol
-
Formation of : Dissolve 3.5 g of in 10 mL water, then add 4 g of in 50 mL water dropwise. Filter and wash the precipitate .
-
Complexation: Combine 4 g of and 5.5 g of in 100 mL water. Add gradually, stirring until dissolved .
-
Crystallization: Concentrate the solution to 30% volume, cool to 10°C, and add 20 mL ethanol to precipitate green crystals .
Optimization Insights
-
pH Control: Maintaining pH 1–2 during complexation prevents hydrolysis of to .
-
Solvent Effects: Ethanol reduces solubility of the product, enhancing yield (75–80%) .
Alternative Approaches Using Structural Templates
Role of Cationic Templates
Recent studies demonstrate that alkylammonium ions (e.g., ) template the crystallization of this compound complexes. For example, forms under mild hydrothermal conditions (80°C, 12 h) .
Comparative Data Table
Method | Starting Material | Oxidizing Agent | Yield (%) | Purity (% Oxalate) |
---|---|---|---|---|
Oxidation of | 63.9 | 53.2 ± 1.6 | ||
Direct Synthesis | None | 75–80 | 55.1 ± 2.1 | |
Templated Synthesis | None | 85–90 | 57.3 ± 1.2 |
Critical Analysis of Methodologies
Efficiency and Scalability
The direct synthesis method offers higher yields (75–80%) but requires stringent pH control. Conversely, the oxidation route is more scalable for industrial applications due to milder conditions .
Purity Challenges
Residual or ions are common contaminants. Recrystallization from ethanol-water mixtures (1:3 v/v) improves purity to >98% .
"The interplay of stoichiometry and reaction dynamics dictates the success of this compound synthesis, underscoring the need for meticulous procedural adherence."
化学反应分析
Types of Reactions: Iron (III) oxalate undergoes various chemical reactions, including:
Oxidation-Reduction Reactions: this compound can be reduced to iron (II) oxalate under certain conditions.
Substitution Reactions: this compound can form mixed-ligand complexes with other ligands such as phenanthroline, resulting in compounds like Fe(phen)2(ox)⋅2H2O.
Common Reagents and Conditions:
Oxalic Acid: Used in the synthesis of this compound from iron (III) hydroxide.
Photolysis: Utilized in the reduction of this compound to iron (II) oxalate.
Major Products:
Iron (II) Oxalate: Formed during the reduction of this compound.
Carbon Dioxide: Released during the photolysis of this compound.
科学研究应用
Environmental Chemistry
Photochemical Reactions and Aerosol Chemistry
Iron (III) oxalate plays a significant role in atmospheric chemistry, particularly in the photochemical oxidation of organic compounds. Studies indicate that this compound complexes can absorb near-UV radiation, leading to the photodissociation of these complexes into reactive species such as iron (II) and carbon dioxide radicals. This process is crucial for understanding the oxidation pathways of dissolved organic matter in aerosols.
- Case Study: Glycolaldehyde Oxidation
Research has shown that when aqueous droplets containing this compound and glycolaldehyde are irradiated at 365 nm, rapid oxidation occurs, resulting in products like glyoxal and glycolic acid. This indicates the potential of this compound to influence aerosol growth and composition significantly .
Materials Science
Synthesis of Magnetic Materials
This compound is utilized in synthesizing magnetic materials through its unique structural properties. The compound can be converted into iron (II) oxalate, which upon thermal decomposition yields magnetic iron oxide nanoparticles.
- Case Study: Magnetic Nanoparticles
The preparation of oxalate-based iron (III) compounds has been explored for their magnetic properties. These materials have potential applications in data storage and biomedical fields due to their tunable magnetic characteristics .
Metallurgy
Iron Recovery Processes
One of the most promising applications of this compound is in the metallurgical field, particularly in innovative iron-making processes that utilize oxalic acid as a medium for dissolving iron ores.
- Process Overview
The proposed method involves several key steps:- Dissolution: Iron oxides are dissolved using oxalic acid to produce an aqueous solution of this compound.
- Photochemical Reduction: The dissolved iron (III) is then photochemically reduced to form precipitated iron (II) oxalate.
- Pyrolytic Reduction: Finally, the iron (II) oxalate is subjected to pyrolysis to yield metallic iron .
This novel approach not only enhances the efficiency of iron recovery but also aims to minimize carbon emissions associated with traditional methods.
Chemical Properties and Complexation
This compound exhibits interesting chemical properties due to its ability to form stable complexes with various ligands. Its stability and solubility characteristics make it a valuable reagent in various chemical reactions.
作用机制
The mechanism of action of iron (III) oxalate involves several key processes:
Photolysis: Upon exposure to light, this compound undergoes intramolecular electron transfer from oxalate to iron, creating iron (II) complexed by one oxidized and two spectator oxalate ligands.
Proton-Coupled Electron Transfer: In the presence of oxalate, iron dissolution from iron (oxyhydr)oxide minerals occurs via proton-coupled electron transfer, enhancing iron bioavailability.
相似化合物的比较
Magnetic Properties
Iron (III) oxalate displays distinct magnetic behavior compared to other oxalate-bridged iron compounds:
Compound | Magnetic Interaction (J, cm⁻¹) | Inter-Chain Coupling | Spontaneous Order | Reference |
---|---|---|---|---|
Fe₂(C₂O₄)₃·5H₂O | −7.58 | Absent | No | |
Fe(III) oxalate with K⁺ | −5.2 to −6.5 | Present | Yes |
This compound’s antiferromagnetic chains approach the ideal Heisenberg spin-chain model, unlike potassium-containing analogs that exhibit spontaneous magnetic order due to inter-chain interactions .
Thermal Stability and Decomposition
This compound decomposes into amorphous iron oxides, which are advantageous for creating high-surface-area materials, whereas Iron (II) oxalate forms crystalline oxides like Fe₃O₄ .
Solubility and Precursor Utility
The high solubility of this compound facilitates its use in doping HA with Fe³⁺, enhancing surface area (194.5 m²/g vs. 159 m²/g for Fe²⁺-HA) and catalytic activity .
Redox and Photochemical Behavior
This compound can be photoreduced to Fe(II) oxalate under UV light, enabling photocatalytic degradation of organic dyes like Rhodamine B . In contrast, Iron (II) oxalate is prone to oxidation, forming mixed Fe(II)/Fe(III) glycerolates when reacted with glycerol .
Proton Conductivity
This compound-ammonium composites, such as (NH₄)₂[Fe(H₂O)Cl₃(C₂O₄)]·H₂O, exhibit proton conductivity (10⁻⁴–10⁻⁵ S/cm) dependent on hydrogen bond density . Similar behavior is rare in non-ammonium iron oxalates, highlighting the role of counterions in modulating ionic transport.
生物活性
Iron (III) oxalate, represented by the formula , is a coordination complex of iron and oxalic acid. This compound has garnered attention due to its biological activity, particularly in the context of iron metabolism, photochemical reactions, and its role in various biochemical processes. This article explores the biological activity of this compound, highlighting its mechanisms, applications, and relevant case studies.
This compound is characterized by its ability to form stable complexes with oxalate ions. The structure consists of iron ions coordinated by oxalate ligands, creating a polymeric network. The iron(III) ions are typically octahedrally coordinated, with oxalate acting as a bidentate ligand:
- Molecular Formula :
- Molar Mass : Approximately 464.00 g/mol
Biological Functions and Mechanisms
This compound plays a significant role in biological systems, particularly in the mobilization and bioavailability of iron. It is involved in various biochemical pathways that facilitate iron uptake by microorganisms and plants.
1. Iron Mobilization
Iron is an essential nutrient for many organisms, but its availability is often limited due to its low solubility in aerobic environments. This compound can enhance iron solubility through the following mechanisms:
- Reduction to Iron (II) : Iron (III) can be reduced to more soluble iron (II) forms through microbial activity or chemical reactions involving reductants such as ascorbate or other organic acids .
- Siderophore Interaction : In some studies, the presence of oxalate has been shown to synergistically enhance the effectiveness of siderophores—molecules that bind and transport iron—by increasing the solubility of iron from mineral sources .
2. Photochemical Activity
This compound exhibits significant photochemical properties. When exposed to light, it can undergo photolysis, leading to the generation of reactive species that may have biological implications:
- Electron Transfer Reactions : Studies have demonstrated that upon photoexcitation, electron transfer occurs from oxalate to iron, producing reactive intermediates . This process can lead to the formation of free radicals that may participate in oxidative stress responses in biological systems.
Case Study 1: Microbial Iron Reduction
A study investigated the role of Paxillus involutus, a wood-decomposing fungus, in reducing iron(III) to iron(II). The research highlighted how extracellular metabolites secreted by this fungus could facilitate the reduction of solid iron(III) complexes into soluble forms . The findings indicated that:
- The fungus produced specific iron(III)-reducing metabolites.
- These metabolites were crucial for enhancing iron bioavailability in nutrient-poor environments.
Case Study 2: Ultraviolet Photochemical Reactions
Research utilizing time-resolved X-ray absorption spectroscopy examined the photochemical behavior of aqueous solutions of ammonium iron(III) oxalate. The study revealed that upon UV excitation, rapid electron transfer processes occurred within picoseconds, leading to significant changes in the oxidation state of iron . Key findings included:
- Formation of reactive intermediates that could influence biological processes.
- Insights into the mechanisms underlying photochemical reactions involving metal-organic complexes.
Data Summary
The following table summarizes key findings related to the biological activity of this compound:
常见问题
Basic Research Questions
Q. How can the oxalate content in Iron (III) oxalate complexes be quantitatively determined using redox titration?
Redox titration with potassium permanganate (KMnO₄) under acidic conditions is a standard method. Dissolve a known mass of the complex in sulfuric acid (H₂SO₄), heat to 70–80°C, and titrate with standardized KMnO₄ until a persistent pink endpoint is reached. The oxalate (C₂O₄²⁻) reduces MnO₄⁻ to Mn²⁺, allowing stoichiometric calculation of oxalate content via the reaction: . Ensure controlled heating to avoid side reactions and validate results against theoretical stoichiometry .
Q. What experimental steps are critical for synthesizing this compound complexes with high purity?
Dissolve Fe(NO₃)₃·9H₂O and K₂C₂O₄·H₂O in separate aqueous solutions, then combine them under constant stirring. Precipitation of the complex is temperature-dependent: higher temperatures (e.g., 60–80°C) improve crystallinity. Filter and wash the product with cold water to remove excess ions. Characterize purity via elemental analysis, IR spectroscopy (to confirm oxalate coordination), and X-ray diffraction .
Q. How can researchers distinguish this compound from Iron (II) oxalate using spectroscopic methods?
UV-Vis spectroscopy reveals distinct absorption bands: this compound exhibits ligand-to-metal charge transfer (LMCT) bands near 250–300 nm, while Iron (II) complexes show d-d transitions at higher wavelengths (500–600 nm). Complement with Mössbauer spectroscopy to confirm oxidation states via quadrupole splitting and isomer shift values .
Advanced Research Questions
Q. What factors contribute to discrepancies between experimental and theoretical oxalate content in this compound complexes?
Common sources include incomplete dissolution of the complex, side reactions (e.g., premature MnO₄⁻ decomposition), or adsorbed water altering sample mass. Use thermogravimetric analysis (TGA) to quantify hydrated water and validate titration results with ion chromatography for independent oxalate quantification .
Q. How does reaction temperature influence the particle size and purity of battery-grade this compound during synthesis?
Higher temperatures (e.g., 60°C) promote Ostwald ripening, yielding larger particles with lower surface impurities. Statistically designed experiments (e.g., response surface methodology) can optimize parameters: temperature, stirring rate, and reactant concentration. Purity ≥99% is achievable at 70°C with a 1:3 Fe³⁺:C₂O₄²⁻ molar ratio .
Q. What mechanisms drive the photochemical degradation of this compound in photocatalytic systems?
Under UV irradiation, Fe(III) oxalate undergoes ligand-to-metal charge transfer, generating Fe²⁺ and CO₂. The Fe²⁺ reacts with H₂O₂ (from dissolved oxygen) to produce hydroxyl radicals (·OH), enabling oxidative degradation of organic pollutants. Monitor reaction kinetics via UV-Vis spectroscopy and electron paramagnetic resonance (EPR) to detect radical intermediates .
Q. How can computational modeling predict the coordination geometry of this compound in aqueous solutions?
Density functional theory (DFT) simulations using B3LYP/6-31G* basis sets model the octahedral coordination of Fe³⁺ with three bidentate oxalate ligands. Compare calculated IR and Raman spectra with experimental data to validate the geometry. Solvation effects are incorporated via implicit solvent models (e.g., COSMO) .
Q. Methodological Considerations
Q. What statistical approaches are recommended for optimizing synthesis parameters of this compound?
Employ a factorial design (e.g., Box-Behnken or central composite design) to analyze interactions between variables (temperature, pH, reactant ratio). Response variables (yield, particle size) are modeled using regression analysis, with ANOVA verifying significance. Validate optimized conditions through triplicate runs .
Q. How should researchers address inconsistencies in reported redox potentials for this compound systems?
Calibrate electrodes using standard solutions (e.g., quinhydrone) and control ionic strength with a supporting electrolyte (e.g., KCl). Cross-reference cyclic voltammetry data with literature values under identical conditions (pH, temperature). Discrepancies may arise from ligand protonation states or Fe³⁺ hydrolysis .
Q. What protocols ensure reproducibility in synthesizing photoactive this compound for hydrogen generation studies?
Use ammonium iron(III) oxalate for enhanced light sensitivity and stability. Prepare photoreactors with controlled light intensity (e.g., solar simulators at 1.5 AM) and quantify H₂ production via gas chromatography. Normalize results to catalyst loading and irradiance to enable cross-study comparisons .
Q. Data Analysis and Contradictions
Q. How can researchers resolve conflicting data on the thermal decomposition pathways of this compound?
Combine TGA with evolved gas analysis (EGA-MS) to identify decomposition products (CO₂, CO, FeO). Conflicting reports may arise from differing heating rates or atmospheric conditions (N₂ vs. air). For example, anaerobic conditions favor FeO formation, while oxidative environments yield Fe₂O₃ .
Q. Why do spectroscopic studies of this compound sometimes show variable LMCT band intensities?
Band intensity depends on crystallinity and solvent interactions. Use standardized sample preparation (e.g., KBr pellets for IR) and degas solutions to minimize oxygen interference. Compare spectra with computational predictions to isolate experimental artifacts .
属性
IUPAC Name |
iron;oxalic acid | |
---|---|---|
Source | PubChem | |
URL | https://pubchem.ncbi.nlm.nih.gov | |
Description | Data deposited in or computed by PubChem | |
InChI |
InChI=1S/3C2H2O4.2Fe/c3*3-1(4)2(5)6;;/h3*(H,3,4)(H,5,6);; | |
Source | PubChem | |
URL | https://pubchem.ncbi.nlm.nih.gov | |
Description | Data deposited in or computed by PubChem | |
InChI Key |
NVDVHKFBLMHJIF-UHFFFAOYSA-N | |
Source | PubChem | |
URL | https://pubchem.ncbi.nlm.nih.gov | |
Description | Data deposited in or computed by PubChem | |
Canonical SMILES |
C(=O)(C(=O)O)O.C(=O)(C(=O)O)O.C(=O)(C(=O)O)O.[Fe].[Fe] | |
Source | PubChem | |
URL | https://pubchem.ncbi.nlm.nih.gov | |
Description | Data deposited in or computed by PubChem | |
Molecular Formula |
C6H6Fe2O12 | |
Source | PubChem | |
URL | https://pubchem.ncbi.nlm.nih.gov | |
Description | Data deposited in or computed by PubChem | |
Molecular Weight |
381.79 g/mol | |
Source | PubChem | |
URL | https://pubchem.ncbi.nlm.nih.gov | |
Description | Data deposited in or computed by PubChem | |
体外研究产品的免责声明和信息
请注意,BenchChem 上展示的所有文章和产品信息仅供信息参考。 BenchChem 上可购买的产品专为体外研究设计,这些研究在生物体外进行。体外研究,源自拉丁语 "in glass",涉及在受控实验室环境中使用细胞或组织进行的实验。重要的是要注意,这些产品没有被归类为药物或药品,他们没有得到 FDA 的批准,用于预防、治疗或治愈任何医疗状况、疾病或疾病。我们必须强调,将这些产品以任何形式引入人类或动物的身体都是法律严格禁止的。遵守这些指南对确保研究和实验的法律和道德标准的符合性至关重要。