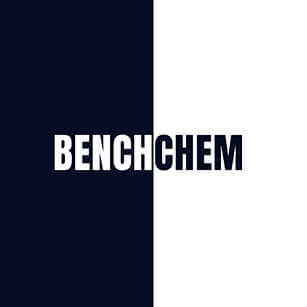
乙炔基苯-13C2
概述
描述
Ethynylbenzene-13C2 is a labeled analogue of ethynylbenzene, an aromatic hydrocarbon. This compound is significant in the petrochemical industry as an intermediate in the production of styrene, which is used to make polystyrene, a common plastic material .
科学研究应用
Ethynylbenzene-13C2 has a wide range of applications in scientific research, including:
Chemistry: Used as a tracer in reaction mechanisms and kinetic studies due to its isotopic labeling.
Biology: Employed in metabolic studies to trace the incorporation of carbon atoms into biological molecules.
Medicine: Utilized in the development of radiolabeled compounds for imaging and diagnostic purposes.
Industry: Serves as an intermediate in the synthesis of various polymers and materials.
作用机制
Target of Action
Ethynylbenzene-13C2, also known as Phenylacetylene-1,2-13C2 , is a labeled analogue of Ethynylbenzene It’s known that ethynylbenzene and its analogues are important in the petrochemical industry as intermediates in the production of styrene .
Mode of Action
The general mechanism of electrophilic aromatic substitution, a common reaction type for aromatic compounds like ethynylbenzene, involves the attack of the pi electrons in the aromatic ring on the electrophile . In the next step, a base attacks the hydrogen, causing the electrons in the C-H bond to form a C-C double bond, and aromaticity is reformed .
Biochemical Pathways
It’s known that ethynylbenzene is used as an intermediate in the production of styrene , which is used for making polystyrene, a common plastic material .
Pharmacokinetics
The compound’s physical properties such as boiling point (142-144 °c) and density (0948 g/mL at 25 °C) are known , which could potentially influence its pharmacokinetic behavior.
Result of Action
As an intermediate in the production of styrene , it contributes to the synthesis of polystyrene, a common plastic material .
准备方法
Synthetic Routes and Reaction Conditions: Ethynylbenzene-13C2 can be synthesized through various methods. One common approach involves the reaction of benzene with acetylene in the presence of a catalyst. The reaction conditions typically include high temperatures and pressures to facilitate the formation of the ethynyl group on the benzene ring .
Industrial Production Methods: In industrial settings, the production of ethynylbenzene-13C2 often involves the use of labeled carbon sources to incorporate the 13C2 isotopes into the ethynyl group. This process ensures the compound’s isotopic labeling, which is crucial for its applications in scientific research .
化学反应分析
Types of Reactions: Ethynylbenzene-13C2 undergoes various chemical reactions, including:
Common Reagents and Conditions:
Oxidation: Potassium permanganate (KMnO4), chromium trioxide (CrO3)
Reduction: Hydrogen gas (H2), palladium catalyst (Pd/C)
Substitution: Bromine (Br2), nitric acid (HNO3)
Major Products Formed:
Oxidation: Benzoic acid
Reduction: Ethylbenzene
Substitution: Brominated or nitrated ethynylbenzene derivatives
相似化合物的比较
Ethynylbenzene-13C2 is unique due to its isotopic labeling, which distinguishes it from other similar compounds. Some similar compounds include:
Ethynylbenzene: The non-labeled analogue, used in similar applications but without the isotopic tracking capability.
Phenylacetylene: Another aromatic compound with an ethynyl group, used in organic synthesis and polymer production.
1-Bromo-2-ethynylbenzene: A brominated derivative used in substitution reactions.
Ethynylbenzene-13C2’s uniqueness lies in its isotopic labeling, making it invaluable for research applications that require precise tracking and analysis of reaction mechanisms .
属性
CAS 编号 |
263012-32-4 |
---|---|
分子式 |
C8H6 |
分子量 |
104.121 |
IUPAC 名称 |
ethynylbenzene |
InChI |
InChI=1S/C8H6/c1-2-8-6-4-3-5-7-8/h1,3-7H/i1+1,2+1 |
InChI 键 |
UEXCJVNBTNXOEH-ZDOIIHCHSA-N |
SMILES |
C#CC1=CC=CC=C1 |
同义词 |
1-Phenylethyne-13C2; Ethynylbenzene-13C2; NSC 4957-13C2; Phenylacetylene-13C2; Phenylethyne-13C2; Ethynyl-1-13C2-benzene; Phenyl[1-13C2]acetylene; |
产品来源 |
United States |
Synthesis routes and methods I
Procedure details
Synthesis routes and methods II
Procedure details
Synthesis routes and methods III
Procedure details
体外研究产品的免责声明和信息
请注意,BenchChem 上展示的所有文章和产品信息仅供信息参考。 BenchChem 上可购买的产品专为体外研究设计,这些研究在生物体外进行。体外研究,源自拉丁语 "in glass",涉及在受控实验室环境中使用细胞或组织进行的实验。重要的是要注意,这些产品没有被归类为药物或药品,他们没有得到 FDA 的批准,用于预防、治疗或治愈任何医疗状况、疾病或疾病。我们必须强调,将这些产品以任何形式引入人类或动物的身体都是法律严格禁止的。遵守这些指南对确保研究和实验的法律和道德标准的符合性至关重要。