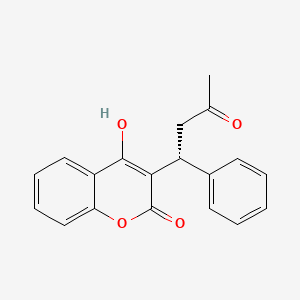
(R)-Warfarin
概述
描述
®-Warfarin is a chiral anticoagulant that is widely used in the prevention and treatment of thromboembolic disorders. It is a derivative of coumarin and functions by inhibiting the synthesis of vitamin K-dependent clotting factors. The compound exists as two enantiomers, ®-Warfarin and (S)-Warfarin, with the (S)-enantiomer being more potent in its anticoagulant activity.
准备方法
Synthetic Routes and Reaction Conditions: The synthesis of ®-Warfarin typically involves the condensation of 4-hydroxycoumarin with benzylideneacetone under basic conditions. The reaction is carried out in the presence of a base such as sodium hydroxide or potassium hydroxide, and the product is isolated through crystallization.
Industrial Production Methods: In industrial settings, the production of ®-Warfarin involves similar synthetic routes but on a larger scale. The process is optimized for yield and purity, often involving additional purification steps such as recrystallization and chromatography to ensure the desired enantiomeric purity.
化学反应分析
Types of Reactions: ®-Warfarin undergoes various chemical reactions, including:
Oxidation: ®-Warfarin can be oxidized to form hydroxy derivatives.
Reduction: The compound can be reduced to form dihydro derivatives.
Substitution: ®-Warfarin can undergo nucleophilic substitution reactions, particularly at the benzylidene moiety.
Common Reagents and Conditions:
Oxidation: Common oxidizing agents include potassium permanganate and chromium trioxide.
Reduction: Reducing agents such as sodium borohydride and lithium aluminum hydride are used.
Substitution: Nucleophiles such as amines and thiols can be used under basic conditions.
Major Products:
Oxidation: Hydroxywarfarin derivatives.
Reduction: Dihydrowarfarin derivatives.
Substitution: Various substituted warfarin derivatives depending on the nucleophile used.
科学研究应用
®-Warfarin has a wide range of applications in scientific research:
Chemistry: It is used as a model compound in studies of chiral separation and enantioselective synthesis.
Biology: ®-Warfarin is used to study the mechanisms of blood coagulation and the role of vitamin K in this process.
Medicine: It is extensively used in clinical research to develop new anticoagulant therapies and to understand the pharmacokinetics and pharmacodynamics of anticoagulants.
Industry: ®-Warfarin is used in the production of rodenticides and as a reference standard in quality control laboratories.
作用机制
®-Warfarin exerts its anticoagulant effects by inhibiting the enzyme vitamin K epoxide reductase (VKOR). This inhibition prevents the conversion of vitamin K epoxide to its active form, thereby reducing the synthesis of vitamin K-dependent clotting factors (II, VII, IX, and X). The reduction in these clotting factors leads to a decrease in blood coagulation.
相似化合物的比较
(S)-Warfarin: The more potent enantiomer of warfarin with stronger anticoagulant activity.
Coumarin: The parent compound from which warfarin is derived.
Dicoumarol: Another anticoagulant that functions similarly to warfarin but with different pharmacokinetic properties.
Uniqueness of ®-Warfarin: ®-Warfarin is unique in its chiral nature and its specific interaction with VKOR. While (S)-Warfarin is more potent, ®-Warfarin has a longer half-life and different metabolic pathways, making it an important compound in the study of anticoagulant therapy and chiral pharmacology.
属性
IUPAC Name |
4-hydroxy-3-[(1R)-3-oxo-1-phenylbutyl]chromen-2-one | |
---|---|---|
Source | PubChem | |
URL | https://pubchem.ncbi.nlm.nih.gov | |
Description | Data deposited in or computed by PubChem | |
InChI |
InChI=1S/C19H16O4/c1-12(20)11-15(13-7-3-2-4-8-13)17-18(21)14-9-5-6-10-16(14)23-19(17)22/h2-10,15,21H,11H2,1H3/t15-/m1/s1 | |
Source | PubChem | |
URL | https://pubchem.ncbi.nlm.nih.gov | |
Description | Data deposited in or computed by PubChem | |
InChI Key |
PJVWKTKQMONHTI-OAHLLOKOSA-N | |
Source | PubChem | |
URL | https://pubchem.ncbi.nlm.nih.gov | |
Description | Data deposited in or computed by PubChem | |
Canonical SMILES |
CC(=O)CC(C1=CC=CC=C1)C2=C(C3=CC=CC=C3OC2=O)O | |
Source | PubChem | |
URL | https://pubchem.ncbi.nlm.nih.gov | |
Description | Data deposited in or computed by PubChem | |
Isomeric SMILES |
CC(=O)C[C@H](C1=CC=CC=C1)C2=C(C3=CC=CC=C3OC2=O)O | |
Source | PubChem | |
URL | https://pubchem.ncbi.nlm.nih.gov | |
Description | Data deposited in or computed by PubChem | |
Molecular Formula |
C19H16O4 | |
Source | PubChem | |
URL | https://pubchem.ncbi.nlm.nih.gov | |
Description | Data deposited in or computed by PubChem | |
Molecular Weight |
308.3 g/mol | |
Source | PubChem | |
URL | https://pubchem.ncbi.nlm.nih.gov | |
Description | Data deposited in or computed by PubChem | |
CAS No. |
5543-58-8, 40281-89-8 | |
Record name | (R)-Warfarin | |
Source | CAS Common Chemistry | |
URL | https://commonchemistry.cas.org/detail?cas_rn=5543-58-8 | |
Description | CAS Common Chemistry is an open community resource for accessing chemical information. Nearly 500,000 chemical substances from CAS REGISTRY cover areas of community interest, including common and frequently regulated chemicals, and those relevant to high school and undergraduate chemistry classes. This chemical information, curated by our expert scientists, is provided in alignment with our mission as a division of the American Chemical Society. | |
Explanation | The data from CAS Common Chemistry is provided under a CC-BY-NC 4.0 license, unless otherwise stated. | |
Record name | (R)-Warfarin | |
Source | ChemIDplus | |
URL | https://pubchem.ncbi.nlm.nih.gov/substance/?source=chemidplus&sourceid=0005543588 | |
Description | ChemIDplus is a free, web search system that provides access to the structure and nomenclature authority files used for the identification of chemical substances cited in National Library of Medicine (NLM) databases, including the TOXNET system. | |
Record name | (R)-warfarin | |
Source | DrugBank | |
URL | https://www.drugbank.ca/drugs/DB08496 | |
Description | The DrugBank database is a unique bioinformatics and cheminformatics resource that combines detailed drug (i.e. chemical, pharmacological and pharmaceutical) data with comprehensive drug target (i.e. sequence, structure, and pathway) information. | |
Explanation | Creative Common's Attribution-NonCommercial 4.0 International License (http://creativecommons.org/licenses/by-nc/4.0/legalcode) | |
Record name | (R)-4-hydroxy-3-(3-oxo-1-phenylbutyl)-2-benzopyrone | |
Source | European Chemicals Agency (ECHA) | |
URL | https://echa.europa.eu/substance-information/-/substanceinfo/100.024.463 | |
Description | The European Chemicals Agency (ECHA) is an agency of the European Union which is the driving force among regulatory authorities in implementing the EU's groundbreaking chemicals legislation for the benefit of human health and the environment as well as for innovation and competitiveness. | |
Explanation | Use of the information, documents and data from the ECHA website is subject to the terms and conditions of this Legal Notice, and subject to other binding limitations provided for under applicable law, the information, documents and data made available on the ECHA website may be reproduced, distributed and/or used, totally or in part, for non-commercial purposes provided that ECHA is acknowledged as the source: "Source: European Chemicals Agency, http://echa.europa.eu/". Such acknowledgement must be included in each copy of the material. ECHA permits and encourages organisations and individuals to create links to the ECHA website under the following cumulative conditions: Links can only be made to webpages that provide a link to the Legal Notice page. | |
Record name | WARFARIN, (R)- | |
Source | FDA Global Substance Registration System (GSRS) | |
URL | https://gsrs.ncats.nih.gov/ginas/app/beta/substances/09CC5J5C8A | |
Description | The FDA Global Substance Registration System (GSRS) enables the efficient and accurate exchange of information on what substances are in regulated products. Instead of relying on names, which vary across regulatory domains, countries, and regions, the GSRS knowledge base makes it possible for substances to be defined by standardized, scientific descriptions. | |
Explanation | Unless otherwise noted, the contents of the FDA website (www.fda.gov), both text and graphics, are not copyrighted. They are in the public domain and may be republished, reprinted and otherwise used freely by anyone without the need to obtain permission from FDA. Credit to the U.S. Food and Drug Administration as the source is appreciated but not required. | |
Synthesis routes and methods I
Procedure details
Synthesis routes and methods II
Procedure details
Q1: What is the primary target of (R)-warfarin?
A1: Like its enantiomer, (S)-warfarin, this compound targets Vitamin K epoxide reductase complex 1 (VKORC1). []
Q2: How does the inhibition of VKORC1 by this compound impact coagulation?
A2: Inhibition of VKORC1 disrupts the vitamin K cycle, which is essential for the γ-carboxylation of vitamin K-dependent clotting factors II, VII, IX, and X, ultimately leading to reduced blood clotting. [, ]
Q3: Is the potency of this compound comparable to (S)-warfarin?
A3: No, this compound exhibits significantly lower anticoagulant activity compared to (S)-warfarin. Studies suggest that (S)-warfarin is 2–5 times more potent than its (R)-enantiomer. []
Q4: What is the molecular formula of this compound?
A4: The molecular formula of this compound is C19H16O4.
Q5: What is the molecular weight of this compound?
A5: The molecular weight of this compound is 308.33 g/mol.
Q6: Are there significant differences in the spectroscopic data between this compound and (S)-warfarin?
A6: While both enantiomers share the same molecular formula and weight, their interactions with chiral environments, such as chiral stationary phases in chromatography, result in distinct spectroscopic behaviors, enabling their differentiation and individual analysis. [, , , , ]
Q7: Does this compound exhibit any inherent catalytic properties?
A8: this compound itself does not possess catalytic activity. Its primary mechanism of action involves binding and inhibiting the enzyme VKORC1, rather than directly catalyzing chemical reactions. [, ]
Q8: Have there been computational studies investigating the interaction of this compound with its target?
A8: While not explicitly detailed in the provided research, computational methods like molecular docking and molecular dynamics simulations are frequently employed to investigate drug-target interactions, providing valuable insights into binding modes and affinities. These techniques can be applied to this compound to understand its binding to VKORC1.
Q9: How does the stereochemistry at the C9 position of warfarin affect its interaction with CYP2C9?
A10: Research suggests that the stereochemistry at the C9 position significantly influences the interaction of warfarin with CYP2C9. Specifically, the ring-closed form of (S)-warfarin exhibits a preferential interaction with CYP2C9, leading to its predominant metabolism. []
Q10: Does the C9 stereocenter influence the anticancer activity of warfarin neoglycosides?
A11: Surprisingly, research on warfarin neoglycosides suggests that the C9 stereocenter does not significantly affect their anticancer activity. Both (R)- and (S)-warfarin-derived neoglycosides display comparable potency against various cancer cell lines. []
Q11: What are the typical formulations of warfarin for clinical use?
A12: Warfarin is typically administered orally as a racemic mixture of (R)- and (S)-enantiomers in the form of tablets. []
Q12: How does the pharmacokinetic profile of this compound differ from (S)-warfarin?
A13: this compound generally exhibits a longer half-life and lower clearance compared to (S)-warfarin. This difference in pharmacokinetic behavior can be attributed to the stereoselective metabolism of warfarin enantiomers by CYP enzymes, particularly CYP2C9. [, , , ]
Q13: Is the clearance of this compound affected by age?
A14: Yes, studies indicate that both total and free clearance of this compound decrease with age, suggesting a potential need for dose adjustments in elderly patients. []
Q14: Does the presence of the low-dose VKORC1*2 haplotype influence the plasma ratio of (S)-warfarin to this compound?
A16: Yes, patients with the VKORC12 haplotype exhibit a significantly different warfarin S/R ratio in their plasma compared to patients carrying high-dose haplotypes (VKORC13 or VKORC1*4). This difference is independent of CYP2C9 variants, suggesting the involvement of other factors linked to VKORC1 haplotypes. []
Q15: What is the impact of fluconazole on the metabolism of this compound in vivo?
A17: Fluconazole, an antifungal agent, potently inhibits the metabolism of this compound, primarily by suppressing CYP3A4 and other CYP isoforms responsible for its clearance. This interaction leads to a significant increase in the plasma concentration and duration of action of this compound. [, ]
Q16: What in vitro models are used to study the metabolism of warfarin enantiomers?
A18: Human liver microsomes are commonly used as an in vitro model to investigate the metabolism of warfarin enantiomers. These microsomes contain the necessary CYP enzymes, particularly CYP2C9, which are responsible for the biotransformation of warfarin. [, , , , ]
Q17: How does the metabolic profile of this compound differ from (S)-warfarin in human liver microsomes?
A19: (S)-Warfarin is primarily metabolized by CYP2C9 to (S)-7-hydroxywarfarin in human liver microsomes, whereas this compound undergoes metabolism by multiple CYP enzymes, including CYP3A4, leading to the formation of various hydroxylated metabolites. []
Q18: Can in vitro data predict in vivo drug interactions involving warfarin metabolism?
A20: Yes, research indicates a strong correlation between in vitro data obtained from human liver microsomes and in vivo drug interactions affecting warfarin metabolism. Specifically, the inhibitory effects of compounds like fluconazole on (S)-warfarin metabolism observed in microsomal studies accurately predict the in vivo interaction, leading to increased warfarin exposure and enhanced anticoagulant effects. [, ]
Q19: Are there reported cases of warfarin resistance related to altered metabolism of this compound?
A21: Yes, there are documented instances of warfarin resistance linked to exceptionally high clearance of (S)-warfarin. In such cases, despite requiring significantly higher warfarin doses to achieve therapeutic anticoagulation, the metabolism of this compound remains largely unaffected. []
Q20: Does this compound contribute to the overall toxicity profile of racemic warfarin?
A20: While both (R)- and (S)-warfarin contribute to the overall anticoagulant effect, the specific contribution of this compound to the toxicity profile of racemic warfarin requires further investigation.
Q21: What analytical techniques are commonly employed for the separation and quantification of warfarin enantiomers?
A23: High-performance liquid chromatography (HPLC) coupled with chiral stationary phases is a widely used technique for separating and quantifying (R)- and (S)-warfarin enantiomers. This method provides the sensitivity and selectivity required for accurate measurement of warfarin enantiomer concentrations in biological samples. [, , , , ]
Q22: Can mass spectrometry be used in conjunction with HPLC for warfarin enantiomer analysis?
A24: Yes, mass spectrometry (MS), particularly electrospray ionization mass spectrometry (ESI-MS), can be coupled with HPLC to further enhance the selectivity and sensitivity of warfarin enantiomer analysis. This combination allows for the identification and quantification of warfarin enantiomers even in complex biological matrices. []
体外研究产品的免责声明和信息
请注意,BenchChem 上展示的所有文章和产品信息仅供信息参考。 BenchChem 上可购买的产品专为体外研究设计,这些研究在生物体外进行。体外研究,源自拉丁语 "in glass",涉及在受控实验室环境中使用细胞或组织进行的实验。重要的是要注意,这些产品没有被归类为药物或药品,他们没有得到 FDA 的批准,用于预防、治疗或治愈任何医疗状况、疾病或疾病。我们必须强调,将这些产品以任何形式引入人类或动物的身体都是法律严格禁止的。遵守这些指南对确保研究和实验的法律和道德标准的符合性至关重要。