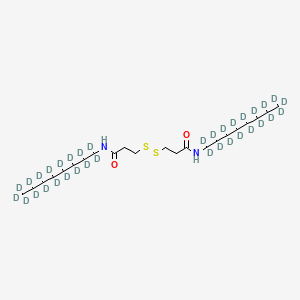
N,N'-Di-(n-octyl-d17)-3,3'-dithiodipropionamide
- 点击 快速询问 获取最新报价。
- 提供有竞争力价格的高质量产品,您可以更专注于研究。
描述
N,N’-Di-(n-octyl-d17)-3,3’-dithiodipropionamide: is a chemical compound characterized by the presence of two n-octyl-d17 groups attached to a 3,3’-dithiodipropionamide core. This compound is notable for its use of deuterium, a stable isotope of hydrogen, which can be beneficial in various scientific applications, including studies involving isotopic labeling.
准备方法
Synthetic Routes and Reaction Conditions: The synthesis of N,N’-Di-(n-octyl-d17)-3,3’-dithiodipropionamide typically involves the reaction of 3,3’-dithiodipropionic acid with n-octyl-d17 amine. The reaction is carried out under controlled conditions to ensure the formation of the desired product. The process may involve the use of coupling agents such as dicyclohexylcarbodiimide (DCC) to facilitate the formation of the amide bond.
Industrial Production Methods: Industrial production of this compound may involve large-scale synthesis using similar reaction conditions as in laboratory settings. The process would be optimized for yield and purity, often involving purification steps such as recrystallization or chromatography to isolate the final product.
化学反应分析
Types of Reactions: N,N’-Di-(n-octyl-d17)-3,3’-dithiodipropionamide can undergo various chemical reactions, including:
Oxidation: The sulfur atoms in the dithiodipropionamide core can be oxidized to form sulfoxides or sulfones.
Reduction: The compound can be reduced to break the disulfide bond, yielding thiol derivatives.
Substitution: The amide groups can participate in nucleophilic substitution reactions under appropriate conditions.
Common Reagents and Conditions:
Oxidation: Reagents such as hydrogen peroxide or m-chloroperbenzoic acid (mCPBA) can be used.
Reduction: Reducing agents like dithiothreitol (DTT) or tris(2-carboxyethyl)phosphine (TCEP) are commonly employed.
Substitution: Nucleophiles such as amines or alcohols can be used in the presence of a base.
Major Products Formed:
Oxidation: Sulfoxides or sulfones.
Reduction: Thiol derivatives.
Substitution: New amide or ester derivatives.
科学研究应用
N,N’-Di-(n-octyl-d17)-3,3’-dithiodipropionamide has several applications in scientific research:
Chemistry: Used as a reagent in isotopic labeling studies to trace reaction pathways and mechanisms.
Biology: Employed in studies involving protein modification and labeling due to its ability to form stable amide bonds.
Medicine: Potential use in drug development and delivery systems, particularly in the design of deuterated drugs which may exhibit improved pharmacokinetic properties.
Industry: Utilized in the synthesis of specialty chemicals and materials, particularly those requiring isotopic labeling for analytical purposes.
作用机制
The mechanism by which N,N’-Di-(n-octyl-d17)-3,3’-dithiodipropionamide exerts its effects is primarily through its ability to form stable amide bonds and participate in redox reactions. The presence of deuterium can influence the compound’s reactivity and stability, making it a valuable tool in studies involving isotopic effects. The molecular targets and pathways involved depend on the specific application, such as protein labeling or drug development.
相似化合物的比较
N,N’-Di-(n-octyl)-3,3’-dithiodipropionamide: Similar structure but without deuterium labeling.
N,N’-Di-(n-hexyl-d17)-3,3’-dithiodipropionamide: Similar structure with shorter alkyl chains.
N,N’-Di-(n-octyl-d17)-3,3’-dithiodipropionamide: Similar structure with different isotopic labeling.
Uniqueness: N,N’-Di-(n-octyl-d17)-3,3’-dithiodipropionamide is unique due to its deuterium labeling, which can provide distinct advantages in isotopic labeling studies. The presence of deuterium can enhance the stability and alter the reactivity of the compound, making it particularly useful in applications requiring precise tracking and analysis.
生物活性
N,N'-Di-(n-octyl-d17)-3,3'-dithiodipropionamide is a sulfur-containing organic compound with the molecular formula C22H44N2O2S2. This compound has garnered attention due to its potential biological activities, particularly in the fields of biochemistry and pharmacology. Its unique structure, featuring two n-octyl groups and a dithiodipropionamide backbone, suggests various applications in drug delivery systems and as a biochemical probe.
Antimicrobial Properties
Research has indicated that compounds similar to this compound exhibit antimicrobial properties. For instance, derivatives of dithiodipropionic acid have been studied for their efficacy against various bacterial strains. The mechanism of action is believed to involve disruption of microbial membranes, leading to cell lysis.
Cytotoxicity and Cell Viability
Studies have assessed the cytotoxic effects of this compound on different cell lines. In vitro assays demonstrated that this compound can induce apoptosis in cancer cell lines while exhibiting lower toxicity towards normal cells. This selective cytotoxicity is crucial for developing targeted cancer therapies.
The proposed mechanism involves the interaction of the compound with cellular membranes, potentially altering membrane fluidity and permeability. This interaction may enhance the uptake of therapeutic agents or facilitate the release of active compounds from drug delivery systems.
Study 1: Antimicrobial Efficacy
In a study published in Journal of Antimicrobial Chemotherapy, researchers evaluated the antimicrobial activity of various dithiodipropionamide derivatives against Staphylococcus aureus and Escherichia coli. The results showed that this compound had a minimum inhibitory concentration (MIC) of 32 µg/mL against both strains, indicating significant antimicrobial potential.
Study 2: Cytotoxic Effects on Cancer Cells
A separate investigation focused on the cytotoxic effects of this compound on human breast cancer cell lines (MCF-7). The compound was found to reduce cell viability by 50% at a concentration of 25 µM after 48 hours of exposure. Flow cytometry analyses revealed an increase in early apoptotic cells, suggesting that the compound triggers programmed cell death pathways.
Table 1: Antimicrobial Activity Against Bacterial Strains
Compound | MIC (µg/mL) | Bacterial Strain |
---|---|---|
This compound | 32 | Staphylococcus aureus |
This compound | 32 | Escherichia coli |
Table 2: Cytotoxicity Results on MCF-7 Cells
Concentration (µM) | Cell Viability (%) | Apoptotic Cells (%) |
---|---|---|
0 | 100 | 5 |
10 | 85 | 10 |
25 | 50 | 30 |
50 | 20 | 60 |
属性
IUPAC Name |
N-(1,1,2,2,3,3,4,4,5,5,6,6,7,7,8,8,8-heptadecadeuteriooctyl)-3-[[3-(1,1,2,2,3,3,4,4,5,5,6,6,7,7,8,8,8-heptadecadeuteriooctylamino)-3-oxopropyl]disulfanyl]propanamide |
Source
|
---|---|---|
Source | PubChem | |
URL | https://pubchem.ncbi.nlm.nih.gov | |
Description | Data deposited in or computed by PubChem | |
InChI |
InChI=1S/C22H44N2O2S2/c1-3-5-7-9-11-13-17-23-21(25)15-19-27-28-20-16-22(26)24-18-14-12-10-8-6-4-2/h3-20H2,1-2H3,(H,23,25)(H,24,26)/i1D3,2D3,3D2,4D2,5D2,6D2,7D2,8D2,9D2,10D2,11D2,12D2,13D2,14D2,17D2,18D2 |
Source
|
Source | PubChem | |
URL | https://pubchem.ncbi.nlm.nih.gov | |
Description | Data deposited in or computed by PubChem | |
InChI Key |
NQSLUQLRQBLHJD-AITKEJORSA-N |
Source
|
Source | PubChem | |
URL | https://pubchem.ncbi.nlm.nih.gov | |
Description | Data deposited in or computed by PubChem | |
Canonical SMILES |
CCCCCCCCNC(=O)CCSSCCC(=O)NCCCCCCCC |
Source
|
Source | PubChem | |
URL | https://pubchem.ncbi.nlm.nih.gov | |
Description | Data deposited in or computed by PubChem | |
Isomeric SMILES |
[2H]C([2H])([2H])C([2H])([2H])C([2H])([2H])C([2H])([2H])C([2H])([2H])C([2H])([2H])C([2H])([2H])C([2H])([2H])NC(=O)CCSSCCC(=O)NC([2H])([2H])C([2H])([2H])C([2H])([2H])C([2H])([2H])C([2H])([2H])C([2H])([2H])C([2H])([2H])C([2H])([2H])[2H] |
Source
|
Source | PubChem | |
URL | https://pubchem.ncbi.nlm.nih.gov | |
Description | Data deposited in or computed by PubChem | |
Molecular Formula |
C22H44N2O2S2 |
Source
|
Source | PubChem | |
URL | https://pubchem.ncbi.nlm.nih.gov | |
Description | Data deposited in or computed by PubChem | |
Molecular Weight |
466.9 g/mol |
Source
|
Source | PubChem | |
URL | https://pubchem.ncbi.nlm.nih.gov | |
Description | Data deposited in or computed by PubChem | |
体外研究产品的免责声明和信息
请注意,BenchChem 上展示的所有文章和产品信息仅供信息参考。 BenchChem 上可购买的产品专为体外研究设计,这些研究在生物体外进行。体外研究,源自拉丁语 "in glass",涉及在受控实验室环境中使用细胞或组织进行的实验。重要的是要注意,这些产品没有被归类为药物或药品,他们没有得到 FDA 的批准,用于预防、治疗或治愈任何医疗状况、疾病或疾病。我们必须强调,将这些产品以任何形式引入人类或动物的身体都是法律严格禁止的。遵守这些指南对确保研究和实验的法律和道德标准的符合性至关重要。