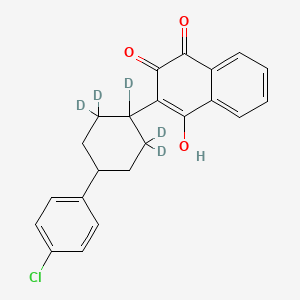
Atovaquone-d5
描述
Atovaquone-d5 is a deuterated analog of atovaquone, a hydroxynaphthoquinone compound. It is primarily used as an antimalarial and antiprotozoal agent. The deuterium labeling in this compound makes it particularly useful in pharmacokinetic studies and as an internal standard in mass spectrometry.
准备方法
Synthetic Routes and Reaction Conditions
The synthesis of atovaquone-d5 involves the incorporation of deuterium atoms into the atovaquone molecule. This can be achieved through various methods, including catalytic hydrogen-deuterium exchange reactions. The reaction typically involves the use of deuterium gas (D2) and a suitable catalyst under controlled conditions to replace hydrogen atoms with deuterium.
Industrial Production Methods
Industrial production of this compound follows similar synthetic routes but on a larger scale. The process involves stringent quality control measures to ensure the purity and consistency of the final product. The use of advanced techniques such as high-performance liquid chromatography (HPLC) and mass spectrometry is common to verify the deuterium incorporation and overall quality of the compound .
化学反应分析
Oxidation Reactions
The hydroxyl group (-OH) attached to the naphthoquinone core undergoes oxidation to form quinone derivatives. Common oxidizing agents include:
-
Potassium permanganate (KMnO₄) in acidic or alkaline conditions.
-
Chromium trioxide (CrO₃) under controlled environments.
For example, oxidation of the hydroxyl group yields fully oxidized naphthoquinone derivatives, which retain the deuterated cyclohexyl substituent .
Reduction Reactions
The quinone moiety can be reduced to hydroquinone (dihydroxynaphthalene) using:
-
Sodium borohydride (NaBH₄) in protic solvents.
Reduction alters the redox-active properties of the molecule, potentially influencing its antimalarial activity.
Electrophilic Substitution Reactions
The aromatic rings in Atovaquone-d5 participate in electrophilic substitution, particularly at the naphthoquinone and chlorophenyl groups. Key reactions include:
-
Bromination : Using bromine (Br₂) in the presence of a Lewis catalyst.
-
Nitration : With nitric acid (HNO₃) under sulfuric acid catalysis .
Substitution reactions typically occur at positions ortho or para to existing electron-withdrawing groups (e.g., chlorine).
Synthetic Routes and Deuterium Incorporation
This compound is synthesized via isotope exchange reactions , replacing hydrogen atoms with deuterium. Methods include:
-
Catalytic hydrogen-deuterium exchange using deuterium gas (D₂) and palladium catalysts .
-
Stepwise deuteration during intermediate synthesis to ensure selective labeling .
Table 1: Key Reaction Conditions and Products
Reaction Type | Reagents/Conditions | Major Products |
---|---|---|
Oxidation | KMnO₄ (acidic), CrO₃ | Oxidized quinone derivatives |
Reduction | NaBH₄ (ethanol), LiAlH₄ (THF) | Hydroquinone analogs |
Bromination | Br₂ (FeBr₃ catalyst) | Brominated naphthoquinone |
Deuteration | D₂ gas, Pd/C catalyst | Deuterated cyclohexyl intermediates |
Stability and Environmental Influences
-
Solubility : Reactivity is influenced by solubility in chloroform, DMSO, or ethyl acetate .
-
pH Sensitivity : The hydroxyl group’s acidity (pKa ≈ 5.01) affects redox behavior in aqueous environments .
Research Findings
-
Biochemical Interactions : The quinone moiety’s redox activity underpins its inhibition of the cytochrome bc1 complex in Plasmodium falciparum . Resistance mutations (e.g., Y268S) reduce hydrophobic interactions, altering binding dynamics .
-
Synthetic Optimization : Industrial production employs HPLC and mass spectrometry to verify deuterium incorporation (>99% purity) .
科学研究应用
Pharmacokinetic Studies
Atovaquone-d5 is extensively utilized in pharmacokinetic studies to provide insights into the behavior of Atovaquone within biological systems. Its deuterium labeling allows for precise tracking in mass spectrometry analyses.
Study Focus | Findings |
---|---|
Absorption | Rapid absorption with peak plasma concentrations reached within 2-4 hours. |
Distribution | Volume of distribution approximately 0.6 L/kg. |
Metabolism | Identified metabolites include hydroxy-atovaquone and others. |
Metabolic Studies
Research involving this compound has elucidated metabolic pathways critical for understanding drug interactions and resistance mechanisms.
- Metabolite Identification : Studies have shown that Atovaquone undergoes extensive metabolism, producing several metabolites that can be traced using this compound as an internal standard .
Drug Development
This compound plays a crucial role in the development of new antimalarial drugs. Its stability and traceability make it an ideal candidate for use in preclinical and clinical trials.
Drug Combination | Efficacy |
---|---|
Atovaquone + Proguanil | High cure rates observed in clinical settings . |
New Antiparasitic Agents | Used as a reference standard to evaluate new compounds. |
Biomedical Research
This compound is investigated for its potential anticancer properties, particularly its effects on mitochondrial function and apoptosis induction in cancer cells.
- Cancer Research Findings : In vitro studies have demonstrated that this compound induces apoptosis in breast cancer cell lines, highlighting its potential as an anticancer agent .
Case Study 1: Antimalarial Efficacy
A clinical study evaluated the efficacy of Atovaquone in combination with Proguanil against Plasmodium falciparum malaria. The combination therapy resulted in a cure rate exceeding 97% among treated patients, showcasing the effectiveness of Atovaquone when used alongside other antimalarials .
Case Study 2: Anticancer Properties
In laboratory settings, this compound was tested against various breast cancer cell lines. Results indicated significant growth inhibition and apoptosis induction, suggesting its potential role as an adjunct therapy in cancer treatment .
作用机制
Atovaquone-d5, like atovaquone, exerts its effects by inhibiting the mitochondrial electron transport chain in parasites. This inhibition disrupts the synthesis of nucleic acids and ATP, leading to the death of the parasite. The primary molecular target is the cytochrome bc1 complex, which is crucial for the mitochondrial function of the parasite .
相似化合物的比较
Atovaquone-d5 is compared with other similar compounds such as:
Ubiquinone: Both are quinone derivatives, but this compound has a higher specificity for parasitic mitochondria.
Lawsone: Another hydroxynaphthoquinone, but this compound is more effective in inhibiting the electron transport chain.
This compound’s uniqueness lies in its deuterium labeling, which enhances its utility in research applications, particularly in mass spectrometry and pharmacokinetic studies.
生物活性
Atovaquone-d5 is a deuterated analog of atovaquone, primarily recognized for its role in treating various parasitic infections, including malaria and Pneumocystis pneumonia. This article delves into the biological activity of this compound, exploring its mechanisms of action, pharmacokinetics, resistance patterns, and potential therapeutic applications.
This compound retains the core structure of atovaquone, which is a hydroxynaphthoquinone. Its mechanism of action is primarily linked to its inhibition of the cytochrome bc1 complex (Complex III) in the mitochondrial electron transport chain. This inhibition disrupts ATP synthesis and leads to a collapse of the mitochondrial membrane potential in susceptible parasites such as Plasmodium falciparum and Pneumocystis carinii .
Target Organism | Mechanism of Action |
---|---|
Plasmodium falciparum | Inhibition of cytochrome bc1 complex |
Pneumocystis carinii | Unknown mechanism; potential mitochondrial effects |
Toxoplasma gondii | Inhibition of mitochondrial processes |
Pharmacokinetics
This compound exhibits similar pharmacokinetic properties to atovaquone, characterized by high lipophilicity and extensive protein binding (over 99.9%). The bioavailability is notably low and variable, significantly influenced by dietary factors. When taken with food, bioavailability can increase to approximately 47%, compared to 23% when fasting .
Table 2: Pharmacokinetic Parameters
Parameter | Value |
---|---|
Bioavailability (with food) | ~47% |
Bioavailability (fasting) | ~23% |
Volume of Distribution | 0.60 ± 0.17 L/kg |
Half-life | 2.2 to 3.2 days |
Clearance | 10.4 ± 5.5 ml/min |
Resistance Patterns
Resistance to this compound has been documented, particularly in P. falciparum, where point mutations in the cytochrome b gene are implicated. These mutations can lead to treatment failures when atovaquone is used as monotherapy . The introduction of combination therapies, such as atovaquone-proguanil (AP), has been a strategic response to mitigate resistance development .
Case Study: Resistance in Malaria Treatment
A notable case study involved three patients who exhibited resistance to atovaquone during treatment for severe malaria. Genetic analysis revealed mutations in the cytochrome b gene, which correlated with treatment failure despite standard dosing regimens . This highlights the necessity for ongoing surveillance and potential adjustments in treatment protocols.
Clinical Applications and Efficacy
This compound demonstrates significant efficacy against a range of protozoan parasites:
- Malaria : Effective against asexual stages of P. falciparum.
- Pneumocystis pneumonia : Used as a prophylactic and therapeutic agent.
- Toxoplasmosis : Shows good in vitro activity.
The recommended dosing regimen for malaria treatment involves a total daily dose of 1500 mg, administered in two divided doses for three days . For prophylaxis against malaria, it is advised to initiate treatment one or two days prior to exposure and continue for seven days post-exposure.
Adverse Effects
This compound is generally well tolerated; however, some adverse reactions have been reported, including gastrointestinal symptoms such as nausea and abdominal pain. Importantly, it does not exhibit significant myelosuppressive effects, making it suitable for immunocompromised patients .
常见问题
Basic Research Questions
Q. What are the validated protocols for synthesizing and characterizing Atovaquone-d5 with isotopic purity?
- Methodological Answer : Synthesis of deuterated compounds like this compound typically involves hydrogen-deuterium exchange reactions or deuterated precursor incorporation. Isotopic purity (>98%) is confirmed via high-resolution mass spectrometry (HRMS) and nuclear magnetic resonance (NMR) spectroscopy, particularly -NMR for deuterium positional verification . Chromatographic techniques (e.g., HPLC with UV detection) are used to assess chemical purity, while isotopic distribution is validated using LC-MS/MS with deuterium-specific fragmentation patterns .
Q. How can researchers evaluate the stability of this compound under varying experimental conditions (e.g., pH, temperature)?
- Methodological Answer : Stability studies should follow ICH guidelines for forced degradation: expose the compound to acidic/alkaline hydrolysis, oxidative stress (HO), thermal stress (40–60°C), and photolytic conditions. Monitor degradation products using stability-indicating HPLC or UPLC methods with diode-array detection (DAD). Kinetic modeling (e.g., Arrhenius plots) quantifies degradation rates, while NMR and MS/MS confirm structural integrity post-stress .
Q. What analytical techniques are optimal for quantifying this compound in biological matrices during preclinical studies?
- Methodological Answer : LC-MS/MS with deuterated internal standards (e.g., this compound itself as an IS) ensures precision. Sample preparation involves protein precipitation (acetonitrile) or solid-phase extraction (SPE) to minimize matrix effects. Calibration curves (1–1000 ng/mL) validated for linearity (R > 0.99), accuracy (85–115%), and intra-/inter-day precision (<15% RSD) are critical. Cross-validate with ELISA or radiometric assays for cross-matrix consistency .
Advanced Research Questions
Q. How can researchers resolve contradictions in reported efficacy data for this compound across different Plasmodium strains?
- Methodological Answer : Conduct comparative dose-response assays (IC) using synchronized parasite cultures under standardized O tension and nutrient conditions. Employ genomic sequencing to identify strain-specific mutations in cytochrome b (target of Atovaquone). Validate findings with isogenic parasite lines engineered via CRISPR-Cas9 to express mutant vs. wild-type cytb. Statistical analysis (ANOVA with post-hoc Tukey tests) quantifies inter-strain variability .
Q. What experimental designs are recommended to study this compound’s mitochondrial toxicity in mammalian cell lines?
- Methodological Answer : Use Seahorse XF analyzers to measure oxygen consumption rate (OCR) and extracellular acidification rate (ECAR) in HepG2 or primary hepatocytes. Dose cells with this compound (0.1–100 µM) and compare to non-deuterated controls. Confirm mitochondrial membrane depolarization via JC-1 staining and ATP quantification (luciferase assays). RNA-seq or proteomics can identify off-target effects on electron transport chain (ETC) complexes .
Q. How can researchers address discrepancies in this compound’s pharmacokinetic (PK) profiles between rodent and non-human primate models?
- Methodological Answer : Perform allometric scaling with species-specific physiological parameters (e.g., liver blood flow, cytochrome P450 expression). Use non-compartmental analysis (NCA) to compute AUC, C, and t in both models. Validate with PBPK modeling (e.g., GastroPlus®) incorporating tissue partition coefficients and enzyme kinetics. Cross-species microdosing studies (C-labeled compound) enhance translational relevance .
Q. What strategies mitigate resistance development to this compound in longitudinal in vivo studies?
- Methodological Answer : Combine this compound with proguanil (to target dihydrofolate reductase) in a fixed-dose regimen. Monitor resistance via weekly blood smears and cytb mutation PCR. Use adaptive dosing (escalating concentrations) in SCID mice engrafted with human erythrocytes. Metagenomic sequencing of parasite populations pre- and post-treatment identifies resistance mechanisms .
Q. Methodological Considerations
- Data Reproducibility : Replicate experiments across ≥3 independent batches, with blinding and randomization to reduce bias. Use open-source tools (e.g., Jupyter Notebooks) for transparent data processing pipelines .
- Ethical Compliance : Adhere to ARRIVE 2.0 guidelines for in vivo studies, including sample size justification and humane endpoints .
属性
IUPAC Name |
3-[4-(4-chlorophenyl)-1,2,2,6,6-pentadeuteriocyclohexyl]-4-hydroxynaphthalene-1,2-dione | |
---|---|---|
Source | PubChem | |
URL | https://pubchem.ncbi.nlm.nih.gov | |
Description | Data deposited in or computed by PubChem | |
InChI |
InChI=1S/C22H19ClO3/c23-16-11-9-14(10-12-16)13-5-7-15(8-6-13)19-20(24)17-3-1-2-4-18(17)21(25)22(19)26/h1-4,9-13,15,24H,5-8H2/i7D2,8D2,15D | |
Source | PubChem | |
URL | https://pubchem.ncbi.nlm.nih.gov | |
Description | Data deposited in or computed by PubChem | |
InChI Key |
BSJMWHQBCZFXBR-YNUDWXFUSA-N | |
Source | PubChem | |
URL | https://pubchem.ncbi.nlm.nih.gov | |
Description | Data deposited in or computed by PubChem | |
Canonical SMILES |
C1CC(CCC1C2=CC=C(C=C2)Cl)C3=C(C4=CC=CC=C4C(=O)C3=O)O | |
Source | PubChem | |
URL | https://pubchem.ncbi.nlm.nih.gov | |
Description | Data deposited in or computed by PubChem | |
Isomeric SMILES |
[2H]C1(CC(CC(C1([2H])C2=C(C3=CC=CC=C3C(=O)C2=O)O)([2H])[2H])C4=CC=C(C=C4)Cl)[2H] | |
Source | PubChem | |
URL | https://pubchem.ncbi.nlm.nih.gov | |
Description | Data deposited in or computed by PubChem | |
Molecular Formula |
C22H19ClO3 | |
Source | PubChem | |
URL | https://pubchem.ncbi.nlm.nih.gov | |
Description | Data deposited in or computed by PubChem | |
DSSTOX Substance ID |
DTXSID10675642 | |
Record name | 3-[4-(4-Chlorophenyl)(1,2,2,6,6-~2~H_5_)cyclohexyl]-4-hydroxynaphthalene-1,2-dione | |
Source | EPA DSSTox | |
URL | https://comptox.epa.gov/dashboard/DTXSID10675642 | |
Description | DSSTox provides a high quality public chemistry resource for supporting improved predictive toxicology. | |
Molecular Weight |
371.9 g/mol | |
Source | PubChem | |
URL | https://pubchem.ncbi.nlm.nih.gov | |
Description | Data deposited in or computed by PubChem | |
CAS No. |
1217612-80-0 | |
Record name | 3-[4-(4-Chlorophenyl)(1,2,2,6,6-~2~H_5_)cyclohexyl]-4-hydroxynaphthalene-1,2-dione | |
Source | EPA DSSTox | |
URL | https://comptox.epa.gov/dashboard/DTXSID10675642 | |
Description | DSSTox provides a high quality public chemistry resource for supporting improved predictive toxicology. | |
体外研究产品的免责声明和信息
请注意,BenchChem 上展示的所有文章和产品信息仅供信息参考。 BenchChem 上可购买的产品专为体外研究设计,这些研究在生物体外进行。体外研究,源自拉丁语 "in glass",涉及在受控实验室环境中使用细胞或组织进行的实验。重要的是要注意,这些产品没有被归类为药物或药品,他们没有得到 FDA 的批准,用于预防、治疗或治愈任何医疗状况、疾病或疾病。我们必须强调,将这些产品以任何形式引入人类或动物的身体都是法律严格禁止的。遵守这些指南对确保研究和实验的法律和道德标准的符合性至关重要。