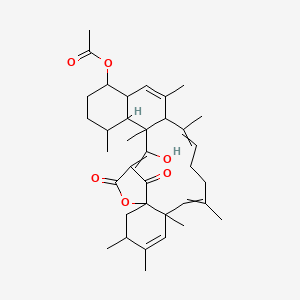
四环霉素A
描述
科学研究应用
作用机制
四环霉素A的作用机制涉及抑制细菌II型拓扑异构酶,如DNA螺旋酶和拓扑异构酶IV . 这些酶对 DNA 复制、转录和染色体分离至关重要。 通过与酶-DNA 复合物结合并使其稳定,四环霉素 A 阻止了 DNA 切割-重连接循环的重新连接步骤,导致 DNA 过程被打乱,最终导致细菌细胞死亡 .
生化分析
Biochemical Properties
Tetromycin A interacts with bacterial type II topoisomerases, such as DNA gyrase and topoisomerase IV . These enzymes are critical for DNA replication, transcription, and chromosomal segregation, as they manage the topology of DNA during these processes . Tetromycin A effectively prevents the re-ligation step of the DNA cleavage-relegation cycle, leading to disruptions in DNA processes .
Cellular Effects
Tetromycin A exerts its effects on various types of cells by disrupting DNA processes, which ultimately leads to bacterial cell death . It has pronounced activity against Gram-positive bacteria, including MRSA . This suggests that Tetromycin A influences cell function by impacting cell signaling pathways, gene expression, and cellular metabolism.
Molecular Mechanism
The mechanism of action of Tetromycin A involves the inhibition of bacterial type II topoisomerases . By binding to and stabilizing the enzyme-DNA complex, Tetromycin A effectively prevents the re-ligation step of the DNA cleavage-relegation cycle . This leads to disruptions in DNA processes at the molecular level, including changes in gene expression .
Temporal Effects in Laboratory Settings
While specific studies detailing the temporal effects of Tetromycin A in laboratory settings are limited, it is known that Tetromycin A derivatives show antiparasitic activities against Trypanosoma brucei and time-dependent inhibition of cathepsin L-like proteases .
Metabolic Pathways
Tetromycin A is a complex polyketide-derived compound .
Transport and Distribution
Given its molecular mechanism of action, it is likely that Tetromycin A interacts with transporters or binding proteins that facilitate its interaction with bacterial type II topoisomerases .
Subcellular Localization
Given its mechanism of action, it is likely that Tetromycin A localizes to areas of the cell where bacterial type II topoisomerases are active .
准备方法
化学反应分析
相似化合物的比较
属性
IUPAC Name |
(23-hydroxy-3,4,6,8,12,14,20,22-octamethyl-25,27-dioxo-26-oxapentacyclo[22.2.1.01,6.013,22.016,21]heptacosa-4,7,11,14,23-pentaen-17-yl) acetate | |
---|---|---|
Source | PubChem | |
URL | https://pubchem.ncbi.nlm.nih.gov | |
Description | Data deposited in or computed by PubChem | |
InChI |
InChI=1S/C36H48O6/c1-19-11-10-12-20(2)29-22(4)15-26-27(41-25(7)37)14-13-21(3)30(26)35(29,9)31(38)28-32(39)36(42-33(28)40)18-24(6)23(5)17-34(36,8)16-19/h12,15-17,21,24,26-27,29-30,38H,10-11,13-14,18H2,1-9H3 | |
Source | PubChem | |
URL | https://pubchem.ncbi.nlm.nih.gov | |
Description | Data deposited in or computed by PubChem | |
InChI Key |
MQEFCZZPPWNMPU-UHFFFAOYSA-N | |
Source | PubChem | |
URL | https://pubchem.ncbi.nlm.nih.gov | |
Description | Data deposited in or computed by PubChem | |
Canonical SMILES |
CC1CCC(C2C1C3(C(C(=CCCC(=CC4(C=C(C(CC45C(=O)C(=C3O)C(=O)O5)C)C)C)C)C)C(=C2)C)C)OC(=O)C | |
Source | PubChem | |
URL | https://pubchem.ncbi.nlm.nih.gov | |
Description | Data deposited in or computed by PubChem | |
Molecular Formula |
C36H48O6 | |
Source | PubChem | |
URL | https://pubchem.ncbi.nlm.nih.gov | |
Description | Data deposited in or computed by PubChem | |
Molecular Weight |
576.8 g/mol | |
Source | PubChem | |
URL | https://pubchem.ncbi.nlm.nih.gov | |
Description | Data deposited in or computed by PubChem | |
Retrosynthesis Analysis
AI-Powered Synthesis Planning: Our tool employs the Template_relevance Pistachio, Template_relevance Bkms_metabolic, Template_relevance Pistachio_ringbreaker, Template_relevance Reaxys, Template_relevance Reaxys_biocatalysis model, leveraging a vast database of chemical reactions to predict feasible synthetic routes.
One-Step Synthesis Focus: Specifically designed for one-step synthesis, it provides concise and direct routes for your target compounds, streamlining the synthesis process.
Accurate Predictions: Utilizing the extensive PISTACHIO, BKMS_METABOLIC, PISTACHIO_RINGBREAKER, REAXYS, REAXYS_BIOCATALYSIS database, our tool offers high-accuracy predictions, reflecting the latest in chemical research and data.
Strategy Settings
Precursor scoring | Relevance Heuristic |
---|---|
Min. plausibility | 0.01 |
Model | Template_relevance |
Template Set | Pistachio/Bkms_metabolic/Pistachio_ringbreaker/Reaxys/Reaxys_biocatalysis |
Top-N result to add to graph | 6 |
Feasible Synthetic Routes
Q1: How do Tetromycins interact with their targets and what are the downstream effects?
A1: The research primarily focuses on the anti-trypanosomal and protease inhibitory activities of the newly discovered Tetromycins []. While the exact mechanism for anti-trypanosomal activity isn't detailed, the study demonstrates these compounds exhibit activity against Trypanosoma brucei. Additionally, the research shows that these Tetromycins act as time-dependent inhibitors of cathepsin L-like proteases, with Ki values in the low micromolar range []. This inhibition suggests potential interference with parasite proteases crucial for their life cycle or infectivity.
体外研究产品的免责声明和信息
请注意,BenchChem 上展示的所有文章和产品信息仅供信息参考。 BenchChem 上可购买的产品专为体外研究设计,这些研究在生物体外进行。体外研究,源自拉丁语 "in glass",涉及在受控实验室环境中使用细胞或组织进行的实验。重要的是要注意,这些产品没有被归类为药物或药品,他们没有得到 FDA 的批准,用于预防、治疗或治愈任何医疗状况、疾病或疾病。我们必须强调,将这些产品以任何形式引入人类或动物的身体都是法律严格禁止的。遵守这些指南对确保研究和实验的法律和道德标准的符合性至关重要。