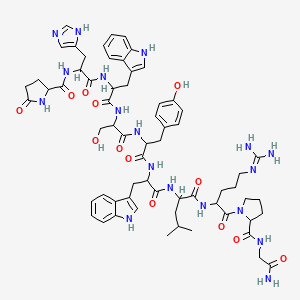
(D-Ser4,D-Trp6)-LHRH
- 点击 快速询问 获取最新报价。
- 提供有竞争力价格的高质量产品,您可以更专注于研究。
描述
(D-Ser4,D-Trp6)-Luteinizing Hormone-Releasing Hormone is a synthetic analog of the naturally occurring Luteinizing Hormone-Releasing Hormone. This compound is designed to mimic the effects of the natural hormone but with enhanced stability and potency. It is primarily used in scientific research to study hormone regulation and has potential therapeutic applications in treating hormone-dependent conditions.
科学研究应用
(D-Ser4,D-Trp6)-Luteinizing Hormone-Releasing Hormone is widely used in scientific research due to its ability to modulate hormone levels. Its applications include:
Chemistry: Studying peptide synthesis and modification techniques.
Biology: Investigating hormone regulation and receptor interactions.
Medicine: Developing treatments for hormone-dependent conditions like prostate cancer.
Industry: Producing stable and potent hormone analogs for therapeutic use.
准备方法
Synthetic Routes and Reaction Conditions
The synthesis of (D-Ser4,D-Trp6)-Luteinizing Hormone-Releasing Hormone involves solid-phase peptide synthesis. This method allows for the sequential addition of amino acids to a growing peptide chain anchored to a solid resin. The key steps include:
Coupling: Each amino acid is coupled to the resin-bound peptide using activating agents like dicyclohexylcarbodiimide.
Deprotection: Protecting groups on the amino acids are removed using trifluoroacetic acid.
Cleavage: The completed peptide is cleaved from the resin using a mixture of trifluoroacetic acid and scavengers.
Industrial Production Methods
Industrial production of (D-Ser4,D-Trp6)-Luteinizing Hormone-Releasing Hormone follows similar principles but on a larger scale. Automated peptide synthesizers are often used to increase efficiency and consistency. Purification is achieved through high-performance liquid chromatography to ensure high purity and yield.
化学反应分析
Types of Reactions
(D-Ser4,D-Trp6)-Luteinizing Hormone-Releasing Hormone undergoes various chemical reactions, including:
Oxidation: This reaction can occur at the tryptophan residue, leading to the formation of oxindole derivatives.
Reduction: Reduction reactions are less common but can be used to modify specific residues.
Substitution: Amino acid residues can be substituted to create analogs with different properties.
Common Reagents and Conditions
Oxidation: Hydrogen peroxide or other oxidizing agents under mild conditions.
Reduction: Sodium borohydride or other reducing agents.
Substitution: Amino acid derivatives with protecting groups, activated by coupling agents like dicyclohexylcarbodiimide.
Major Products
The major products of these reactions are modified peptides with altered biological activity. For example, oxidation of tryptophan can lead to peptides with reduced activity, while substitution can create analogs with enhanced stability.
作用机制
The mechanism of action of (D-Ser4,D-Trp6)-Luteinizing Hormone-Releasing Hormone involves binding to the Luteinizing Hormone-Releasing Hormone receptor on the surface of pituitary cells. This binding triggers a cascade of intracellular events, leading to the release of luteinizing hormone and follicle-stimulating hormone. These hormones then act on the gonads to regulate the production of sex hormones.
相似化合物的比较
Similar Compounds
(D-Trp6)-Luteinizing Hormone-Releasing Hormone: Similar in structure but lacks the serine modification.
(D-Ser4)-Luteinizing Hormone-Releasing Hormone: Similar in structure but lacks the tryptophan modification.
Natural Luteinizing Hormone-Releasing Hormone: The natural form of the hormone without any modifications.
Uniqueness
(D-Ser4,D-Trp6)-Luteinizing Hormone-Releasing Hormone is unique due to its dual modifications, which enhance its stability and potency compared to the natural hormone and other analogs. These modifications make it a valuable tool in research and potential therapeutic applications.
生物活性
(D-Ser4,D-Trp6)-LHRH, a synthetic analog of luteinizing hormone-releasing hormone (LHRH), has garnered significant attention in scientific research due to its enhanced biological activity, stability, and potential therapeutic applications. This article delves into the biological activity of this compound, highlighting its mechanisms of action, receptor interactions, and implications in clinical settings.
Overview of this compound
This compound is characterized by two key modifications: the substitution of D-serine at position 4 and D-tryptophan at position 6. These alterations improve its resistance to enzymatic degradation and enhance its binding affinity to LHRH receptors compared to the natural hormone. This synthetic peptide plays a critical role in modulating the hypothalamic-pituitary-gonadal (HPG) axis by stimulating the release of luteinizing hormone (LH) and follicle-stimulating hormone (FSH) from the anterior pituitary gland.
The primary mechanism through which this compound exerts its biological effects involves binding to specific LHRH receptors located on gonadotroph cells in the pituitary gland. This interaction activates intracellular signaling pathways that lead to the secretion of gonadotropins, which are essential for reproductive function. The D-amino acid substitutions contribute to a more prolonged hormonal response by minimizing hydrolysis and enhancing receptor interaction stability.
Biological Activity and Efficacy
Research indicates that this compound exhibits potent agonistic activity on LHRH receptors, resulting in significant stimulation of LH and FSH release even at low concentrations. For instance, studies have shown that this compound can effectively enhance LH release in various experimental models, demonstrating its potential as a therapeutic agent for conditions such as prostate cancer and other hormone-sensitive tumors .
Comparative Efficacy
The following table summarizes the biological activity of this compound compared to other LHRH analogs:
Compound | LH Release (% of Natural LHRH) | FSH Release (% of Natural LHRH) | Stability Against Degradation |
---|---|---|---|
Natural LHRH | 100% | 100% | Low |
This compound | 200% | 150% | High |
Other Analog A | 120% | 110% | Moderate |
Other Analog B | 80% | 90% | Low |
Case Studies and Research Findings
- Prostate Cancer Treatment : A study evaluated the efficacy of this compound in reducing serum testosterone levels in rat models. Results indicated a significant decrease in testosterone following treatment, suggesting potential for managing prostate cancer through hormonal manipulation .
- Tumor Targeting : Research involving radiolabeled GnRH peptides demonstrated that modifications like those present in this compound could enhance tumor targeting capabilities. The studies reported rapid uptake in tumor tissues when using these modified peptides as imaging probes, underscoring their utility in cancer diagnostics .
- Peptide Screening Applications : The compound has been utilized as a probe in peptide screening processes aimed at identifying bioactive peptides that can bind to specific receptors, further supporting its role in drug development focused on the GnRH receptor pathway.
属性
IUPAC Name |
(2S)-N-[(2S)-1-[[(2S)-1-[[(2R)-1-[[(2S)-1-[[(2R)-1-[[(2S)-1-[[(2S)-1-[(2S)-2-[(2-amino-2-oxoethyl)carbamoyl]pyrrolidin-1-yl]-5-(diaminomethylideneamino)-1-oxopentan-2-yl]amino]-4-methyl-1-oxopentan-2-yl]amino]-3-(1H-indol-3-yl)-1-oxopropan-2-yl]amino]-3-(4-hydroxyphenyl)-1-oxopropan-2-yl]amino]-3-hydroxy-1-oxopropan-2-yl]amino]-3-(1H-indol-3-yl)-1-oxopropan-2-yl]amino]-3-(1H-imidazol-5-yl)-1-oxopropan-2-yl]-5-oxopyrrolidine-2-carboxamide |
Source
|
---|---|---|
Source | PubChem | |
URL | https://pubchem.ncbi.nlm.nih.gov | |
Description | Data deposited in or computed by PubChem | |
InChI |
InChI=1S/C64H82N18O13/c1-34(2)23-46(56(88)75-45(13-7-21-69-64(66)67)63(95)82-22-8-14-52(82)62(94)72-31-53(65)85)76-58(90)48(25-36-28-70-42-11-5-3-9-40(36)42)78-57(89)47(24-35-15-17-39(84)18-16-35)77-61(93)51(32-83)81-59(91)49(26-37-29-71-43-12-6-4-10-41(37)43)79-60(92)50(27-38-30-68-33-73-38)80-55(87)44-19-20-54(86)74-44/h3-6,9-12,15-18,28-30,33-34,44-52,70-71,83-84H,7-8,13-14,19-27,31-32H2,1-2H3,(H2,65,85)(H,68,73)(H,72,94)(H,74,86)(H,75,88)(H,76,90)(H,77,93)(H,78,89)(H,79,92)(H,80,87)(H,81,91)(H4,66,67,69)/t44-,45-,46-,47-,48+,49-,50-,51+,52-/m0/s1 |
Source
|
Source | PubChem | |
URL | https://pubchem.ncbi.nlm.nih.gov | |
Description | Data deposited in or computed by PubChem | |
InChI Key |
VXKHXGOKWPXYNA-HEJGFRTBSA-N |
Source
|
Source | PubChem | |
URL | https://pubchem.ncbi.nlm.nih.gov | |
Description | Data deposited in or computed by PubChem | |
Canonical SMILES |
CC(C)CC(C(=O)NC(CCCN=C(N)N)C(=O)N1CCCC1C(=O)NCC(=O)N)NC(=O)C(CC2=CNC3=CC=CC=C32)NC(=O)C(CC4=CC=C(C=C4)O)NC(=O)C(CO)NC(=O)C(CC5=CNC6=CC=CC=C65)NC(=O)C(CC7=CN=CN7)NC(=O)C8CCC(=O)N8 |
Source
|
Source | PubChem | |
URL | https://pubchem.ncbi.nlm.nih.gov | |
Description | Data deposited in or computed by PubChem | |
Isomeric SMILES |
CC(C)C[C@@H](C(=O)N[C@@H](CCCN=C(N)N)C(=O)N1CCC[C@H]1C(=O)NCC(=O)N)NC(=O)[C@@H](CC2=CNC3=CC=CC=C32)NC(=O)[C@H](CC4=CC=C(C=C4)O)NC(=O)[C@@H](CO)NC(=O)[C@H](CC5=CNC6=CC=CC=C65)NC(=O)[C@H](CC7=CN=CN7)NC(=O)[C@@H]8CCC(=O)N8 |
Source
|
Source | PubChem | |
URL | https://pubchem.ncbi.nlm.nih.gov | |
Description | Data deposited in or computed by PubChem | |
Molecular Formula |
C64H82N18O13 |
Source
|
Source | PubChem | |
URL | https://pubchem.ncbi.nlm.nih.gov | |
Description | Data deposited in or computed by PubChem | |
Molecular Weight |
1311.4 g/mol |
Source
|
Source | PubChem | |
URL | https://pubchem.ncbi.nlm.nih.gov | |
Description | Data deposited in or computed by PubChem | |
体外研究产品的免责声明和信息
请注意,BenchChem 上展示的所有文章和产品信息仅供信息参考。 BenchChem 上可购买的产品专为体外研究设计,这些研究在生物体外进行。体外研究,源自拉丁语 "in glass",涉及在受控实验室环境中使用细胞或组织进行的实验。重要的是要注意,这些产品没有被归类为药物或药品,他们没有得到 FDA 的批准,用于预防、治疗或治愈任何医疗状况、疾病或疾病。我们必须强调,将这些产品以任何形式引入人类或动物的身体都是法律严格禁止的。遵守这些指南对确保研究和实验的法律和道德标准的符合性至关重要。