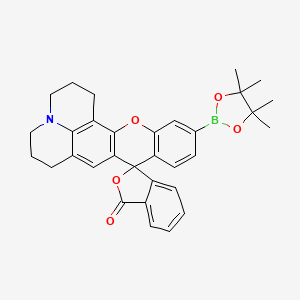
Peroxy Orange 1
- 点击 快速询问 获取最新报价。
- 提供有竞争力价格的高质量产品,您可以更专注于研究。
描述
Peroxy Orange 1 (PO1) is a novel fluorescent probe specifically designed for the detection of hydrogen peroxide (H2O2). It is cell-permeable and displays an orange intracellular fluorescence in response to H2O2 signals produced in cells during immune response and growth factor stimulation . The chemical name of Peroxy Orange 1 is 2’,3’,6’,7’-Tetrahydro-12’-(4,4,5,5-tetramethyl-1,3,2-dioxaborolan-2-yl)-spiro[isobenzofuran-1(3H),9’-[1H,5H,9H]xantheno[2,3,4-ij]quinolizin]-3-one .
Chemical Reactions Analysis
Peroxy Orange 1 is a specific probe for hydrogen peroxide (H2O2). It binds to green fluorescent highly reactive oxygen species (hROS) probe APF . More detailed information about its chemical reactions was not found in the search results.
Physical And Chemical Properties Analysis
Peroxy Orange 1 is soluble up to 10 mM in DMSO . It is a pink solid at room temperature . More detailed physical and chemical properties were not found in the search results.
Relevant Papers
Two relevant papers were found in the search results . The first paper, titled “A palette of fluorescent probes with varying emission colors for imaging hydrogen peroxide signaling in living cells”, discusses the use of Peroxy Orange 1 and other fluorescent probes for imaging hydrogen peroxide signaling in living cells . The second paper, titled “Subcellular localization of the FLT3-ITD oncogene plays a significant role in the production of NOX- and p22phox-derived reactive oxygen species in acute myeloid leukemia”, discusses the role of Peroxy Orange 1 in detecting reactive oxygen species in acute myeloid leukemia .
科学研究应用
Peroxy Orange 1: Scientific Research Applications
1. Detection of Hydrogen Peroxide in Biological Systems Peroxy Orange 1 (PO1) is primarily used as a fluorescent probe for detecting hydrogen peroxide (H2O2) in biological systems. It is highly permeable and non-trappable, making it suitable for real-time monitoring of H2O2 levels in various cellular processes .
Immune Response Monitoring: In immune cells like RAW 264.7 macrophages, PO1 can be used to visualize the production of H2O2 during the immune response, providing insights into the cellular mechanisms underlying inflammation and immunity .
Growth Factor Stimulation Studies: PO1’s ability to display orange intracellular fluorescence in response to H2O2 signals allows researchers to study the role of growth factors in cell signaling pathways, particularly in cells like A431 during growth factor stimulation .
Plant Biology Research: In plant biology, PO1 has been utilized to detect H2O2 in root-to-shoot silencing studies and to understand the oxidative stress responses in different plant mutants .
Live-Cell Imaging: Due to its photochemical properties similar to orange fluorescent proteins (FPs), PO1 is compatible with live-cell imaging techniques, allowing for simultaneous use with green/yellow FPs without interference .
Advanced Gene Editing: PO1 can be employed in advanced gene editing techniques where precise detection of oxidative stress markers like H2O2 is crucial for understanding gene expression and regulation mechanisms .
Materials Science & Engineering: In materials science, PO1’s sensitivity to H2O2 can be leveraged to study oxidative processes in materials engineering, such as corrosion or degradation of materials under oxidative stress .
Microbiological Testing: PO1’s fluorescent properties make it a valuable tool for microbiological testing where detection of oxidative stress responses is necessary for pathogen identification or antibiotic resistance studies .
作用机制
属性
IUPAC Name |
6'-(4,4,5,5-tetramethyl-1,3,2-dioxaborolan-2-yl)spiro[2-benzofuran-3,10'-3-oxa-17-azapentacyclo[11.7.1.02,11.04,9.017,21]henicosa-1(21),2(11),4(9),5,7,12-hexaene]-1-one |
Source
|
---|---|---|
Source | PubChem | |
URL | https://pubchem.ncbi.nlm.nih.gov | |
Description | Data deposited in or computed by PubChem | |
InChI |
InChI=1S/C32H32BNO5/c1-30(2)31(3,4)39-33(38-30)20-13-14-24-26(18-20)36-28-22-11-8-16-34-15-7-9-19(27(22)34)17-25(28)32(24)23-12-6-5-10-21(23)29(35)37-32/h5-6,10,12-14,17-18H,7-9,11,15-16H2,1-4H3 |
Source
|
Source | PubChem | |
URL | https://pubchem.ncbi.nlm.nih.gov | |
Description | Data deposited in or computed by PubChem | |
InChI Key |
KNTNXZHHCPNNGB-UHFFFAOYSA-N |
Source
|
Source | PubChem | |
URL | https://pubchem.ncbi.nlm.nih.gov | |
Description | Data deposited in or computed by PubChem | |
Canonical SMILES |
B1(OC(C(O1)(C)C)(C)C)C2=CC3=C(C=C2)C4(C5=CC=CC=C5C(=O)O4)C6=C(O3)C7=C8C(=C6)CCCN8CCC7 |
Source
|
Source | PubChem | |
URL | https://pubchem.ncbi.nlm.nih.gov | |
Description | Data deposited in or computed by PubChem | |
Molecular Formula |
C32H32BNO5 |
Source
|
Source | PubChem | |
URL | https://pubchem.ncbi.nlm.nih.gov | |
Description | Data deposited in or computed by PubChem | |
Molecular Weight |
521.4 g/mol |
Source
|
Source | PubChem | |
URL | https://pubchem.ncbi.nlm.nih.gov | |
Description | Data deposited in or computed by PubChem | |
Product Name |
Peroxy Orange 1 |
Q & A
Q1: What makes Peroxy Orange 1 a suitable probe for studying biological processes involving hydrogen peroxide?
A1: Peroxy Orange 1 (PO1) offers several advantages as a probe for hydrogen peroxide (H2O2) in biological systems:
- Specificity: PO1 demonstrates high selectivity for H2O2 over other reactive oxygen species (ROS) like hypochlorous acid (HOCl). This specificity is crucial for accurately attributing observed effects to H2O2 signaling. []
- Sensitivity: PO1 exhibits a "turn-on" fluorescence response upon reacting with H2O2, allowing for the detection of physiologically relevant H2O2 concentrations. This sensitivity is essential for monitoring subtle changes in H2O2 levels during cellular signaling events. [, ]
- Live-cell imaging: The fluorescence properties of PO1 are compatible with live-cell imaging techniques, enabling researchers to monitor real-time changes in H2O2 levels within living cells. [, ]
Q2: How does Peroxy Orange 1 detect hydrogen peroxide?
A2: Peroxy Orange 1 employs a boronate-based detection mechanism. The boronate moiety within the probe reacts specifically with H2O2, leading to a structural change that triggers a fluorescence increase. This "turn-on" response allows for the sensitive and selective detection of H2O2. [, ]
Q3: Can you provide examples of how Peroxy Orange 1 has been used to investigate hydrogen peroxide signaling in biological systems?
A3: Peroxy Orange 1 has proven valuable in uncovering new insights into H2O2 signaling:
- Plant Biology: Researchers used PO1 to demonstrate that abscisic acid (ABA), a plant hormone involved in drought response, increases H2O2 levels specifically within the mitochondria of guard cells, ultimately leading to stomatal closure. []
- Immunology: PO1 facilitated the visualization of different H2O2 production patterns within phagosomes of activated macrophages, highlighting the diverse roles of H2O2 in immune responses. []
- Cancer Biology: Studies utilizing PO1 revealed that NADPH oxidase-generated H2O2 contributes to DNA damage in leukemia cells expressing mutant FLT3, a receptor tyrosine kinase often mutated in acute myeloid leukemia. This finding sheds light on potential mechanisms driving aggressive leukemia phenotypes. []
Q4: What are the limitations of using Peroxy Orange 1 for H2O2 detection?
A4: While a powerful tool, PO1 does have some limitations:
Q5: Are there alternative methods for detecting H2O2 in biological systems?
A5: Yes, several alternative methods exist for H2O2 detection, each with advantages and disadvantages:
Q6: What are the future directions for research involving Peroxy Orange 1 and similar probes?
A6: Future research could focus on:
体外研究产品的免责声明和信息
请注意,BenchChem 上展示的所有文章和产品信息仅供信息参考。 BenchChem 上可购买的产品专为体外研究设计,这些研究在生物体外进行。体外研究,源自拉丁语 "in glass",涉及在受控实验室环境中使用细胞或组织进行的实验。重要的是要注意,这些产品没有被归类为药物或药品,他们没有得到 FDA 的批准,用于预防、治疗或治愈任何医疗状况、疾病或疾病。我们必须强调,将这些产品以任何形式引入人类或动物的身体都是法律严格禁止的。遵守这些指南对确保研究和实验的法律和道德标准的符合性至关重要。