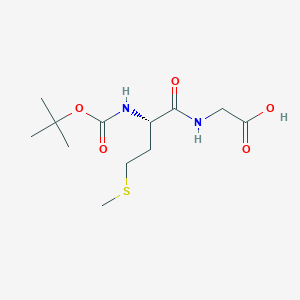
Boc-Met-Gly-OH
描述
Boc-met-gly-OH, also known as N-tert-butoxycarbonyl-L-methionyl-glycine, is a dipeptide compound where the amino acid methionine is protected by a tert-butoxycarbonyl (Boc) group. This compound is commonly used in peptide synthesis and serves as an intermediate in the production of various peptides and proteins. The Boc group is a protecting group that prevents unwanted reactions at the amino group during chemical synthesis.
科学研究应用
Boc-met-gly-OH has a wide range of applications in scientific research, including:
Peptide Synthesis: It is used as an intermediate in the synthesis of peptides and proteins. The Boc group protects the amino group of methionine during peptide bond formation.
Biological Studies: this compound is used in studies involving protein structure and function. It serves as a model compound for understanding peptide interactions and stability.
Medical Research: The compound is used in the development of peptide-based drugs and therapeutic agents. It is also used in the study of enzyme-substrate interactions and protein-ligand binding.
Industrial Applications: this compound is used in the production of peptide-based products such as pharmaceuticals, cosmetics, and food additives.
作用机制
Target of Action
Boc-met-gly-OH is a derivative of amino acids, where Boc stands for tert-butoxycarbonyl, a protective group used in peptide synthesis . The primary targets of this compound are likely to be the same as those of the amino acids methionine and glycine, from which it is derived . These targets can include various enzymes, receptors, and transporters in the body, depending on the specific biochemical context.
Mode of Action
The mode of action of this compound involves its interaction with its targets. As a derivative of amino acids, it may interact with its targets in a similar way to the parent amino acids. The Boc group serves as a protective group, preventing the amino group from reacting until it is removed . This allows for selective reactions to occur at other sites on the molecule. The removal of the Boc group can be achieved under relatively mild conditions, such as with strong acid (trifluoroacetic acid) or heat .
Biochemical Pathways
The exact biochemical pathways affected by this compound would depend on the specific context of its use. As a derivative of amino acids, it could potentially be involved in protein synthesis or other amino acid-related pathways . .
Pharmacokinetics
For example, it could potentially be absorbed in the gut, distributed throughout the body via the bloodstream, metabolized in the liver, and excreted in the urine. The presence of the Boc group may influence these properties, for example by affecting its solubility or stability .
Result of Action
The molecular and cellular effects of this compound’s action would depend on its specific targets and the context of its use. As a derivative of amino acids, it could potentially influence protein structure and function, enzymatic reactions, and cellular signaling pathways . The presence of the Boc group may alter these effects compared to the parent amino acids.
Action Environment
The action, efficacy, and stability of this compound can be influenced by various environmental factors. These can include the pH and temperature of the surrounding environment, the presence of other molecules, and the specific cellular context . For example, the removal of the Boc group can be influenced by the acidity and temperature of the environment .
生化分析
Biochemical Properties
The role of Boc-Met-Gly-OH in biochemical reactions is primarily as a building block in peptide synthesis . It interacts with various enzymes and proteins during the synthesis process. The Boc group in this compound is stable towards most nucleophiles and bases, making it suitable for use in various biochemical reactions .
Cellular Effects
The effects of this compound on cellular processes are largely dependent on the context in which it is used. As a component in peptide synthesis, it can influence cell function by contributing to the formation of proteins that play key roles in cell signaling pathways, gene expression, and cellular metabolism .
Molecular Mechanism
The molecular mechanism of action of this compound involves its participation in peptide bond formation during protein synthesis. It can bind to biomolecules, participate in enzyme activation or inhibition, and contribute to changes in gene expression through the proteins it helps form .
Temporal Effects in Laboratory Settings
The effects of this compound over time in laboratory settings can vary. Its stability, degradation, and long-term effects on cellular function are subject to the specific conditions of the experiment. For instance, the Boc group can be removed under acidic conditions, allowing for further reactions to occur .
Metabolic Pathways
This compound, as a peptide, may be involved in protein metabolism pathways. It could interact with enzymes and cofactors involved in these pathways, potentially affecting metabolic flux or metabolite levels .
Transport and Distribution
The transport and distribution of this compound within cells and tissues would depend on the specific context of its use. In the context of peptide synthesis, it would be transported to the site of protein synthesis and incorporated into the growing peptide chain .
Subcellular Localization
The subcellular localization of this compound would be determined by the protein into which it is incorporated. This could include any targeting signals or post-translational modifications that direct the protein to specific compartments or organelles .
准备方法
Synthetic Routes and Reaction Conditions
The synthesis of Boc-met-gly-OH typically involves the following steps:
Protection of Methionine: Methionine is first protected by reacting it with di-tert-butyl dicarbonate (Boc2O) in the presence of a base such as sodium hydroxide or triethylamine. This reaction forms Boc-methionine.
Coupling with Glycine: Boc-methionine is then coupled with glycine using a coupling reagent such as N,N’-dicyclohexylcarbodiimide (DCC) or N,N’-diisopropylcarbodiimide (DIC) in the presence of a catalyst like 4-dimethylaminopyridine (DMAP) or hydroxybenzotriazole (HOBt). The reaction is typically carried out in an organic solvent such as dichloromethane or dimethylformamide (DMF).
Industrial Production Methods
In an industrial setting, the production of this compound follows similar synthetic routes but on a larger scale. The reactions are optimized for higher yields and purity, and the processes are automated to ensure consistency and efficiency. The use of continuous flow reactors and advanced purification techniques such as high-performance liquid chromatography (HPLC) are common in industrial production.
化学反应分析
Types of Reactions
Boc-met-gly-OH undergoes several types of chemical reactions, including:
Deprotection: The Boc group can be removed under acidic conditions using reagents such as trifluoroacetic acid (TFA) or hydrochloric acid (HCl) in methanol. This reaction yields the free amine form of methionine.
Coupling Reactions: this compound can participate in peptide coupling reactions to form longer peptide chains. This is typically done using coupling reagents like DCC or DIC.
Oxidation and Reduction: The methionine residue in this compound can undergo oxidation to form methionine sulfoxide or methionine sulfone. Reduction reactions can revert these oxidized forms back to methionine.
Common Reagents and Conditions
Deprotection: Trifluoroacetic acid (TFA), hydrochloric acid (HCl)
Coupling: N,N’-dicyclohexylcarbodiimide (DCC), N,N’-diisopropylcarbodiimide (DIC), 4-dimethylaminopyridine (DMAP), hydroxybenzotriazole (HOBt)
Oxidation: Hydrogen peroxide (H2O2), dimethyl sulfoxide (DMSO)
Reduction: Sodium borohydride (NaBH4), dithiothreitol (DTT)
Major Products Formed
Deprotection: Free amine form of methionine
Coupling: Longer peptide chains
Oxidation: Methionine sulfoxide, methionine sulfone
相似化合物的比较
Similar Compounds
Boc-glycine: A single amino acid protected by a Boc group. It is used in peptide synthesis as a building block.
Boc-alanine: Another Boc-protected amino acid used in peptide synthesis.
Boc-phenylalanine: A Boc-protected aromatic amino acid used in the synthesis of peptides and proteins.
Uniqueness of Boc-met-gly-OH
This compound is unique due to its structure as a dipeptide with a Boc-protected methionine residue. This makes it particularly useful in the synthesis of peptides that require methionine as a component. The presence of the glycine residue also adds flexibility to the peptide chain, making it an important intermediate in the synthesis of various peptides and proteins.
属性
IUPAC Name |
2-[[(2S)-2-[(2-methylpropan-2-yl)oxycarbonylamino]-4-methylsulfanylbutanoyl]amino]acetic acid | |
---|---|---|
Source | PubChem | |
URL | https://pubchem.ncbi.nlm.nih.gov | |
Description | Data deposited in or computed by PubChem | |
InChI |
InChI=1S/C12H22N2O5S/c1-12(2,3)19-11(18)14-8(5-6-20-4)10(17)13-7-9(15)16/h8H,5-7H2,1-4H3,(H,13,17)(H,14,18)(H,15,16)/t8-/m0/s1 | |
Source | PubChem | |
URL | https://pubchem.ncbi.nlm.nih.gov | |
Description | Data deposited in or computed by PubChem | |
InChI Key |
PEKPTLWQVLOJAJ-QMMMGPOBSA-N | |
Source | PubChem | |
URL | https://pubchem.ncbi.nlm.nih.gov | |
Description | Data deposited in or computed by PubChem | |
Canonical SMILES |
CC(C)(C)OC(=O)NC(CCSC)C(=O)NCC(=O)O | |
Source | PubChem | |
URL | https://pubchem.ncbi.nlm.nih.gov | |
Description | Data deposited in or computed by PubChem | |
Isomeric SMILES |
CC(C)(C)OC(=O)N[C@@H](CCSC)C(=O)NCC(=O)O | |
Source | PubChem | |
URL | https://pubchem.ncbi.nlm.nih.gov | |
Description | Data deposited in or computed by PubChem | |
Molecular Formula |
C12H22N2O5S | |
Source | PubChem | |
URL | https://pubchem.ncbi.nlm.nih.gov | |
Description | Data deposited in or computed by PubChem | |
Molecular Weight |
306.38 g/mol | |
Source | PubChem | |
URL | https://pubchem.ncbi.nlm.nih.gov | |
Description | Data deposited in or computed by PubChem | |
Retrosynthesis Analysis
AI-Powered Synthesis Planning: Our tool employs the Template_relevance Pistachio, Template_relevance Bkms_metabolic, Template_relevance Pistachio_ringbreaker, Template_relevance Reaxys, Template_relevance Reaxys_biocatalysis model, leveraging a vast database of chemical reactions to predict feasible synthetic routes.
One-Step Synthesis Focus: Specifically designed for one-step synthesis, it provides concise and direct routes for your target compounds, streamlining the synthesis process.
Accurate Predictions: Utilizing the extensive PISTACHIO, BKMS_METABOLIC, PISTACHIO_RINGBREAKER, REAXYS, REAXYS_BIOCATALYSIS database, our tool offers high-accuracy predictions, reflecting the latest in chemical research and data.
Strategy Settings
Precursor scoring | Relevance Heuristic |
---|---|
Min. plausibility | 0.01 |
Model | Template_relevance |
Template Set | Pistachio/Bkms_metabolic/Pistachio_ringbreaker/Reaxys/Reaxys_biocatalysis |
Top-N result to add to graph | 6 |
Feasible Synthetic Routes
体外研究产品的免责声明和信息
请注意,BenchChem 上展示的所有文章和产品信息仅供信息参考。 BenchChem 上可购买的产品专为体外研究设计,这些研究在生物体外进行。体外研究,源自拉丁语 "in glass",涉及在受控实验室环境中使用细胞或组织进行的实验。重要的是要注意,这些产品没有被归类为药物或药品,他们没有得到 FDA 的批准,用于预防、治疗或治愈任何医疗状况、疾病或疾病。我们必须强调,将这些产品以任何形式引入人类或动物的身体都是法律严格禁止的。遵守这些指南对确保研究和实验的法律和道德标准的符合性至关重要。