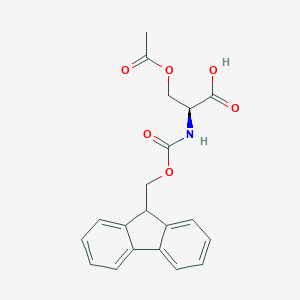
Fmoc-Ser(Ac)-OH
描述
Fmoc-Ser(Ac)-OH, also known as Fmoc-O-acetyl-L-serine, is a derivative of serine . It is used in the preparation of a wide range of virus membrane fusion inhibitors . It is a basic monosaccharide fragment of branched N-linked oligosaccharides found on glycoproteins .
Synthesis Analysis
This compound is synthesized using Fmoc solid-phase peptide synthesis (SPPS) . The O-glycosidic linkage and the O-acetyl protection in this building block are stable to both piperidine and TFA, making it completely compatible with standard protocols in Fmoc solid phase peptide synthesis . Removal of the acetyl-protecting groups is best carried out by treatment of the peptidyl resin with methanolic ammonia prior to cleavage .Molecular Structure Analysis
The molecular formula of this compound is C20H19NO6 . Its molecular weight is 369.4 g/mol . The IUPAC name is (2S)-3-acetyloxy-2-(9H-fluoren-9-ylmethoxycarbonylamino)propanoic acid .Chemical Reactions Analysis
The chemical reactions involving this compound are part of the Fmoc solid-phase peptide synthesis . The O-glycosidic linkage and the O-acetyl protection in this building block are stable to both piperidine and TFA .Physical and Chemical Properties Analysis
This compound has a molecular weight of 369.4 g/mol . It has a XLogP3-AA value of 2.6, indicating its lipophilicity . It has 2 hydrogen bond donors and 6 hydrogen bond acceptors . Its exact mass and monoisotopic mass are 369.12123733 g/mol . Its topological polar surface area is 102 Ų .科学研究应用
作用机制
Target of Action
Fmoc-Ser(Ac)-OH, a derivative of the amino acid serine, is primarily used as a building block in peptide synthesis . The primary targets of this compound are the peptide chains being synthesized, where it contributes to the formation of specific peptide sequences .
Mode of Action
The mode of action of this compound involves its incorporation into peptide chains through a process known as Fmoc/tBu solid-phase synthesis . This synthetic strategy involves a solid polymeric protecting group and allows the use of an excess of reagents to achieve quantitative yields . The Fmoc group serves as a temporary protecting group for the amino group during peptide chain assembly .
Biochemical Pathways
The biochemical pathways involved in the action of this compound are primarily those related to peptide synthesis. The compound plays a key role in the formation of peptide bonds, contributing to the assembly of complex peptide structures .
Pharmacokinetics
Its solubility and stability under various conditions are crucial for its effective use in peptide synthesis .
Result of Action
The result of this compound’s action is the successful synthesis of the desired peptide chains. These peptides can then be used in various applications, from research to the production of therapeutic agents .
Action Environment
The action of this compound is influenced by various environmental factors. For instance, pH and buffer ions play a role in the self-assembly of this compound to gel formation . Additionally, physical and thermal stimuli can be used for solubilizing this compound above the critical concentration to induce gel formation .
生化分析
Biochemical Properties
Fmoc-Ser(Ac)-OH is known for its eminent self-assembly features, which are largely attributed to the inherent hydrophobicity and aromaticity of the Fmoc moiety . This property allows this compound to interact with various enzymes, proteins, and other biomolecules, promoting the association of building blocks .
Cellular Effects
The effects of this compound on cells and cellular processes are diverse. It influences cell function, including impacts on cell signaling pathways, gene expression, and cellular metabolism . The inherent hydrophobicity and aromaticity of the Fmoc moiety in this compound can promote the association of building blocks, which may influence various cellular processes .
Molecular Mechanism
The molecular mechanism of this compound involves its interaction with biomolecules at the molecular level. The Fmoc group in this compound can promote the association of building blocks, potentially influencing enzyme activity, biomolecular binding interactions, and changes in gene expression .
Temporal Effects in Laboratory Settings
In laboratory settings, the effects of this compound can change over time. Factors such as the product’s stability, degradation, and long-term effects on cellular function are important considerations in in vitro or in vivo studies .
属性
IUPAC Name |
(2S)-3-acetyloxy-2-(9H-fluoren-9-ylmethoxycarbonylamino)propanoic acid | |
---|---|---|
Source | PubChem | |
URL | https://pubchem.ncbi.nlm.nih.gov | |
Description | Data deposited in or computed by PubChem | |
InChI |
InChI=1S/C20H19NO6/c1-12(22)26-11-18(19(23)24)21-20(25)27-10-17-15-8-4-2-6-13(15)14-7-3-5-9-16(14)17/h2-9,17-18H,10-11H2,1H3,(H,21,25)(H,23,24)/t18-/m0/s1 | |
Source | PubChem | |
URL | https://pubchem.ncbi.nlm.nih.gov | |
Description | Data deposited in or computed by PubChem | |
InChI Key |
HSGIKRPBGCJRDB-SFHVURJKSA-N | |
Source | PubChem | |
URL | https://pubchem.ncbi.nlm.nih.gov | |
Description | Data deposited in or computed by PubChem | |
Canonical SMILES |
CC(=O)OCC(C(=O)O)NC(=O)OCC1C2=CC=CC=C2C3=CC=CC=C13 | |
Source | PubChem | |
URL | https://pubchem.ncbi.nlm.nih.gov | |
Description | Data deposited in or computed by PubChem | |
Isomeric SMILES |
CC(=O)OC[C@@H](C(=O)O)NC(=O)OCC1C2=CC=CC=C2C3=CC=CC=C13 | |
Source | PubChem | |
URL | https://pubchem.ncbi.nlm.nih.gov | |
Description | Data deposited in or computed by PubChem | |
Molecular Formula |
C20H19NO6 | |
Source | PubChem | |
URL | https://pubchem.ncbi.nlm.nih.gov | |
Description | Data deposited in or computed by PubChem | |
DSSTOX Substance ID |
DTXSID20427038 | |
Record name | Fmoc-L-Ser(Ac) | |
Source | EPA DSSTox | |
URL | https://comptox.epa.gov/dashboard/DTXSID20427038 | |
Description | DSSTox provides a high quality public chemistry resource for supporting improved predictive toxicology. | |
Molecular Weight |
369.4 g/mol | |
Source | PubChem | |
URL | https://pubchem.ncbi.nlm.nih.gov | |
Description | Data deposited in or computed by PubChem | |
CAS No. |
171778-17-9 | |
Record name | O-Acetyl-N-[(9H-fluoren-9-ylmethoxy)carbonyl]-L-serine | |
Source | CAS Common Chemistry | |
URL | https://commonchemistry.cas.org/detail?cas_rn=171778-17-9 | |
Description | CAS Common Chemistry is an open community resource for accessing chemical information. Nearly 500,000 chemical substances from CAS REGISTRY cover areas of community interest, including common and frequently regulated chemicals, and those relevant to high school and undergraduate chemistry classes. This chemical information, curated by our expert scientists, is provided in alignment with our mission as a division of the American Chemical Society. | |
Explanation | The data from CAS Common Chemistry is provided under a CC-BY-NC 4.0 license, unless otherwise stated. | |
Record name | NSC 778228 | |
Source | ChemIDplus | |
URL | https://pubchem.ncbi.nlm.nih.gov/substance/?source=chemidplus&sourceid=0171778179 | |
Description | ChemIDplus is a free, web search system that provides access to the structure and nomenclature authority files used for the identification of chemical substances cited in National Library of Medicine (NLM) databases, including the TOXNET system. | |
Record name | Fmoc-L-Ser(Ac) | |
Source | EPA DSSTox | |
URL | https://comptox.epa.gov/dashboard/DTXSID20427038 | |
Description | DSSTox provides a high quality public chemistry resource for supporting improved predictive toxicology. | |
Retrosynthesis Analysis
AI-Powered Synthesis Planning: Our tool employs the Template_relevance Pistachio, Template_relevance Bkms_metabolic, Template_relevance Pistachio_ringbreaker, Template_relevance Reaxys, Template_relevance Reaxys_biocatalysis model, leveraging a vast database of chemical reactions to predict feasible synthetic routes.
One-Step Synthesis Focus: Specifically designed for one-step synthesis, it provides concise and direct routes for your target compounds, streamlining the synthesis process.
Accurate Predictions: Utilizing the extensive PISTACHIO, BKMS_METABOLIC, PISTACHIO_RINGBREAKER, REAXYS, REAXYS_BIOCATALYSIS database, our tool offers high-accuracy predictions, reflecting the latest in chemical research and data.
Strategy Settings
Precursor scoring | Relevance Heuristic |
---|---|
Min. plausibility | 0.01 |
Model | Template_relevance |
Template Set | Pistachio/Bkms_metabolic/Pistachio_ringbreaker/Reaxys/Reaxys_biocatalysis |
Top-N result to add to graph | 6 |
Feasible Synthetic Routes
体外研究产品的免责声明和信息
请注意,BenchChem 上展示的所有文章和产品信息仅供信息参考。 BenchChem 上可购买的产品专为体外研究设计,这些研究在生物体外进行。体外研究,源自拉丁语 "in glass",涉及在受控实验室环境中使用细胞或组织进行的实验。重要的是要注意,这些产品没有被归类为药物或药品,他们没有得到 FDA 的批准,用于预防、治疗或治愈任何医疗状况、疾病或疾病。我们必须强调,将这些产品以任何形式引入人类或动物的身体都是法律严格禁止的。遵守这些指南对确保研究和实验的法律和道德标准的符合性至关重要。