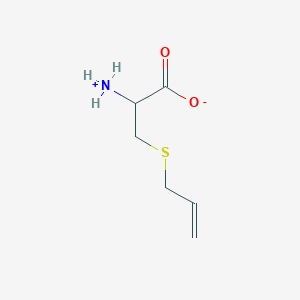
S-烯丙基-L-半胱氨酸
概述
描述
S-allyl-L-cysteine is an organosulfur compound derived from the amino acid cysteine. It is primarily found in aged garlic and is known for its potential medicinal properties. The compound has the chemical formula HO₂CCH(NH₂)CH₂SCH₂CH=CH₂ and is significant biologically in its L-enantiomer form .
科学研究应用
S-allyl-L-cysteine has a wide range of scientific research applications:
作用机制
S-allyl-L-cysteine exerts its effects through several mechanisms:
Antioxidant Activity: It scavenges free radicals and reduces oxidative stress.
Enzyme Inhibition: The compound inhibits enzymes such as calpain, which are involved in cell death pathways.
Gene Expression Modulation: S-allyl-L-cysteine influences the expression of genes related to antioxidant defense and apoptosis.
安全和危害
未来方向
SAC has shown promising results in neurodegenerative disease and there is interest in it as a prototype for developing novel therapeutic drugs for neurological diseases . Further in vivo studies should be carried out to clarify the underlying mechanisms responsible for the neuroprotective effects of SAC .
生化分析
Biochemical Properties
S-allyl-L-cysteine plays a crucial role in several biochemical reactions. It interacts with various enzymes, proteins, and other biomolecules, influencing their activity and function. One of the primary interactions of S-allyl-L-cysteine is with hydrogen sulfide-synthesizing enzymes such as 3-mercaptopyruvate sulfurtransferase, cystathionine γ-lyase, and cystathionine β-synthase . These interactions are essential for the modulation of hydrogen sulfide levels in cells, which in turn affects various physiological processes. Additionally, S-allyl-L-cysteine has been shown to inhibit prooxidant enzymes and chelate metals, contributing to its antioxidant properties .
Cellular Effects
S-allyl-L-cysteine exerts significant effects on various types of cells and cellular processes. In cancer cells, such as MCF-7 and MDA-MB-231 breast adenocarcinoma cell lines, S-allyl-L-cysteine has been shown to reduce cell proliferation and induce apoptosis . This compound also influences cell signaling pathways, including the downregulation of nuclear factor kappa B and the modulation of oxidative stress responses . Furthermore, S-allyl-L-cysteine affects gene expression by altering the expression of enzymes involved in hydrogen sulfide metabolism .
Molecular Mechanism
The molecular mechanism of S-allyl-L-cysteine involves several key interactions at the molecular level. S-allyl-L-cysteine binds to the Ca2±binding domain of calpain, an enzyme involved in neurotoxicity, thereby inhibiting its activity . This binding interaction is crucial for the neuroprotective effects of S-allyl-L-cysteine. Additionally, S-allyl-L-cysteine modulates the expression of genes involved in oxidative stress responses and inflammation, contributing to its antioxidant and anti-inflammatory properties .
Temporal Effects in Laboratory Settings
In laboratory settings, the effects of S-allyl-L-cysteine have been observed to change over time. Studies have shown that S-allyl-L-cysteine remains stable under various conditions, maintaining its biological activity . Long-term exposure to S-allyl-L-cysteine has been associated with sustained neuroprotective effects and the inhibition of oxidative stress-induced cell death . These findings suggest that S-allyl-L-cysteine can provide prolonged therapeutic benefits in in vitro and in vivo models.
Dosage Effects in Animal Models
The effects of S-allyl-L-cysteine vary with different dosages in animal models. At lower doses, S-allyl-L-cysteine has been shown to exert beneficial effects, such as reducing oxidative stress and inflammation . At higher doses, S-allyl-L-cysteine may exhibit toxic effects, including hepatotoxicity and nephrotoxicity . It is essential to determine the optimal dosage to maximize the therapeutic benefits while minimizing adverse effects.
Metabolic Pathways
S-allyl-L-cysteine is involved in several metabolic pathways, including the metabolism of hydrogen sulfide. It interacts with enzymes such as 3-mercaptopyruvate sulfurtransferase, cystathionine γ-lyase, and cystathionine β-synthase, which are involved in the endogenous formation of hydrogen sulfide from L-cysteine . These interactions are crucial for maintaining cellular redox balance and modulating various physiological processes.
Transport and Distribution
Within cells and tissues, S-allyl-L-cysteine is transported and distributed through specific transporters and binding proteins. The compound is known to interact with γ-glutamyl transpeptidase, which plays a role in its transport and distribution . This interaction facilitates the localization and accumulation of S-allyl-L-cysteine in specific cellular compartments, enhancing its biological activity.
Subcellular Localization
S-allyl-L-cysteine exhibits specific subcellular localization, which is essential for its activity and function. Studies have shown that S-allyl-L-cysteine localizes in the cytosol and interacts with various organelles, including the endoplasmic reticulum and mitochondria . This localization is mediated by targeting signals and post-translational modifications that direct S-allyl-L-cysteine to specific compartments, where it exerts its biological effects.
准备方法
Synthetic Routes and Reaction Conditions
S-allyl-L-cysteine can be synthesized through various methods. One common approach involves the reaction of cysteine with allyl bromide under basic conditions. The reaction typically proceeds as follows:
- Dissolve cysteine in water.
- Add a base such as sodium hydroxide to the solution.
- Introduce allyl bromide to the mixture.
- Stir the reaction mixture at room temperature for several hours.
- Purify the product through crystallization or chromatography.
Industrial Production Methods
Industrial production of S-allyl-L-cysteine often involves the extraction from garlic. The process includes:
- Crushing fresh garlic to release its juices.
- Allowing the garlic to age, during which S-allyl-L-cysteine forms naturally.
- Extracting the compound using solvents such as ethanol or water.
- Purifying the extract through filtration and evaporation .
化学反应分析
Types of Reactions
S-allyl-L-cysteine undergoes several types of chemical reactions, including:
Oxidation: It can be oxidized to form sulfoxides and sulfones.
Reduction: The compound can be reduced to form thiols.
Substitution: S-allyl-L-cysteine can participate in nucleophilic substitution reactions, where the allyl group is replaced by other functional groups.
Common Reagents and Conditions
Oxidation: Common oxidizing agents include hydrogen peroxide and potassium permanganate.
Reduction: Reducing agents such as sodium borohydride and lithium aluminum hydride are used.
Substitution: Reagents like alkyl halides and acids are employed under basic or acidic conditions.
Major Products Formed
Oxidation: Sulfoxides and sulfones.
Reduction: Thiols.
Substitution: Various substituted cysteine derivatives.
相似化合物的比较
S-allyl-L-cysteine is unique compared to other similar compounds due to its specific biological activities and stability. Similar compounds include:
S-allylmercaptocysteine: Another organosulfur compound found in garlic, known for its anticancer properties.
γ-glutamyl-S-allylcysteine: A precursor to S-allyl-L-cysteine, also found in garlic.
S-ethyl-L-cysteine: A derivative with similar neuroprotective effects but different potency
S-allyl-L-cysteine stands out due to its well-documented health benefits and its presence in aged garlic, making it a valuable compound for both research and practical applications.
属性
IUPAC Name |
(2R)-2-amino-3-prop-2-enylsulfanylpropanoic acid | |
---|---|---|
Source | PubChem | |
URL | https://pubchem.ncbi.nlm.nih.gov | |
Description | Data deposited in or computed by PubChem | |
InChI |
InChI=1S/C6H11NO2S/c1-2-3-10-4-5(7)6(8)9/h2,5H,1,3-4,7H2,(H,8,9)/t5-/m0/s1 | |
Source | PubChem | |
URL | https://pubchem.ncbi.nlm.nih.gov | |
Description | Data deposited in or computed by PubChem | |
InChI Key |
ZFAHNWWNDFHPOH-YFKPBYRVSA-N | |
Source | PubChem | |
URL | https://pubchem.ncbi.nlm.nih.gov | |
Description | Data deposited in or computed by PubChem | |
Canonical SMILES |
C=CCSCC(C(=O)O)N | |
Source | PubChem | |
URL | https://pubchem.ncbi.nlm.nih.gov | |
Description | Data deposited in or computed by PubChem | |
Isomeric SMILES |
C=CCSC[C@@H](C(=O)O)N | |
Source | PubChem | |
URL | https://pubchem.ncbi.nlm.nih.gov | |
Description | Data deposited in or computed by PubChem | |
Molecular Formula |
C6H11NO2S | |
Source | PubChem | |
URL | https://pubchem.ncbi.nlm.nih.gov | |
Description | Data deposited in or computed by PubChem | |
DSSTOX Substance ID |
DTXSID20894862 | |
Record name | S-Allylcysteine | |
Source | EPA DSSTox | |
URL | https://comptox.epa.gov/dashboard/DTXSID20894862 | |
Description | DSSTox provides a high quality public chemistry resource for supporting improved predictive toxicology. | |
Molecular Weight |
161.22 g/mol | |
Source | PubChem | |
URL | https://pubchem.ncbi.nlm.nih.gov | |
Description | Data deposited in or computed by PubChem | |
CAS RN |
21593-77-1 | |
Record name | (+)-S-Allylcysteine | |
Source | CAS Common Chemistry | |
URL | https://commonchemistry.cas.org/detail?cas_rn=21593-77-1 | |
Description | CAS Common Chemistry is an open community resource for accessing chemical information. Nearly 500,000 chemical substances from CAS REGISTRY cover areas of community interest, including common and frequently regulated chemicals, and those relevant to high school and undergraduate chemistry classes. This chemical information, curated by our expert scientists, is provided in alignment with our mission as a division of the American Chemical Society. | |
Explanation | The data from CAS Common Chemistry is provided under a CC-BY-NC 4.0 license, unless otherwise stated. | |
Record name | S-Allylcysteine | |
Source | ChemIDplus | |
URL | https://pubchem.ncbi.nlm.nih.gov/substance/?source=chemidplus&sourceid=0021593771 | |
Description | ChemIDplus is a free, web search system that provides access to the structure and nomenclature authority files used for the identification of chemical substances cited in National Library of Medicine (NLM) databases, including the TOXNET system. | |
Record name | S-Allylcysteine | |
Source | EPA DSSTox | |
URL | https://comptox.epa.gov/dashboard/DTXSID20894862 | |
Description | DSSTox provides a high quality public chemistry resource for supporting improved predictive toxicology. | |
Record name | S-ALLYLCYSTEINE | |
Source | FDA Global Substance Registration System (GSRS) | |
URL | https://gsrs.ncats.nih.gov/ginas/app/beta/substances/81R3X99M15 | |
Description | The FDA Global Substance Registration System (GSRS) enables the efficient and accurate exchange of information on what substances are in regulated products. Instead of relying on names, which vary across regulatory domains, countries, and regions, the GSRS knowledge base makes it possible for substances to be defined by standardized, scientific descriptions. | |
Explanation | Unless otherwise noted, the contents of the FDA website (www.fda.gov), both text and graphics, are not copyrighted. They are in the public domain and may be republished, reprinted and otherwise used freely by anyone without the need to obtain permission from FDA. Credit to the U.S. Food and Drug Administration as the source is appreciated but not required. | |
Retrosynthesis Analysis
AI-Powered Synthesis Planning: Our tool employs the Template_relevance Pistachio, Template_relevance Bkms_metabolic, Template_relevance Pistachio_ringbreaker, Template_relevance Reaxys, Template_relevance Reaxys_biocatalysis model, leveraging a vast database of chemical reactions to predict feasible synthetic routes.
One-Step Synthesis Focus: Specifically designed for one-step synthesis, it provides concise and direct routes for your target compounds, streamlining the synthesis process.
Accurate Predictions: Utilizing the extensive PISTACHIO, BKMS_METABOLIC, PISTACHIO_RINGBREAKER, REAXYS, REAXYS_BIOCATALYSIS database, our tool offers high-accuracy predictions, reflecting the latest in chemical research and data.
Strategy Settings
Precursor scoring | Relevance Heuristic |
---|---|
Min. plausibility | 0.01 |
Model | Template_relevance |
Template Set | Pistachio/Bkms_metabolic/Pistachio_ringbreaker/Reaxys/Reaxys_biocatalysis |
Top-N result to add to graph | 6 |
Feasible Synthetic Routes
Q & A
ANone: [] SAC can alleviate oxidative stress-induced injuries in chondrocytes, likely by downregulating genes involved in the Mitochondrial Inflammation Pathway (MIP). This includes genes like hypoxia-inducible factor 1α (Hif-1α), Xanthine Oxidase (XO), Caspase-9 (Casp-9), Caspase-3 (Casp-3), Interleukin 1 beta (IL-1β), and inducible nitric oxide synthase (iNOS). This downregulation leads to improved cell viability, morphology, and cell migration. Additionally, it decreases lactate dehydrogenase release, increases superoxide dismutase release, and enhances glycosaminoglycans retention. (Ref: S-ALLYL-L-CYSTEINE-INDUCED ANTI-INFLAMMATORY AND ANTI-APOPTOTIC EFFECTS IN CHONDROCYTES IS ASSOCIATED WITH SUPPRESSION OF THE MITOCHONDRIAL INFLAMMATION PATHWAY)
ANone: [] Yes, SAC has shown the ability to induce cell proliferation in specific contexts. For example, in primary cultures of adult rat hepatocytes, SAC stimulated time- and dose-dependent proliferation. This effect was linked to the autocrine secretion of Insulin-like Growth Factor 1 (IGF-1) triggered by SAC. The secreted IGF-1 subsequently activates the IGF-1 Receptor Tyrosine Kinase (RTK)/PI3K/MEK/ERK/mTOR pathway, ultimately leading to hepatocyte proliferation. (Ref: Signal transduction mechanism for S-allyl-L-cysteine-induced cell proliferation in primary cultures of adult rat hepatocytes)
ANone: [] The molecular formula of SAC is C6H11NO2S, and its molecular weight is 161.22 g/mol. (Ref: Isolation of (—)S-Allyl-L-cysteine from Garlic)
ANone: [] Yes, studies have utilized various spectroscopic techniques to characterize SAC. For instance, researchers employed optical rotatory dispersion (ORD) and circular dichroism (CD) to analyze the diastereomers of SAC S-oxides. These studies revealed the significant influence of the allyl group on the chiroptical properties of the sulfoxides. (Ref: Optical rotatory dispersion and circular dichroism of diastereomeric S-allyl-L-cysteine S-oxides)
ANone: [] The stability of SAC is influenced by cooking methods and conditions. While blanching and microwaving show minimal impact on SAC content, boiling and autoclaving lead to significant increases. Elevated temperatures and prolonged cooking times further amplify this increase in SAC content. (Ref: 마늘의 조리방법에 따른 S-Allyl-L-Cysteine 함량 변화)
ANone: [] While SAC itself is not an enzyme, it acts as a substrate for various enzymes. Notably, flavin-containing monooxygenases (FMOs) can catalyze the S-oxidation of SAC to its corresponding sulfoxide. The specific FMO isoform involved often depends on the structure of the cysteine conjugate. (Ref: Oxidation of cysteine S-conjugates by rabbit liver microsomes and cDNA-expressed flavin-containing mono-oxygenases: studies with S-(1,2-dichlorovinyl)-L-cysteine, S-(1,2,2-trichlorovinyl)-L-cysteine, S-allyl-L-cysteine, and S-benzyl-L-cysteine.)
ANone: [] SAC exhibits high oral bioavailability, exceeding 90%. It undergoes N-acetylation and S-oxidation during metabolism, and its elimination involves extensive renal reabsorption. The liver and kidneys play crucial roles in the elimination of SAC. (Ref: Metabolism, Excretion, and Pharmacokinetics of S-Allyl-l-Cysteine in Rats and Dogs)
ANone: [] SAC demonstrates neuroprotective properties, particularly in cerebral ischemic injury models. It has been shown to reduce infarct size following transient or global ischemic insults. This effect is attributed to its ability to scavenge peroxynitrite (ONOO−) and inhibit the activity of extracellular signal-regulated kinase (ERK). (Ref: S-Allyl-l-cysteine attenuates cerebral ischemic injury by scavenging peroxynitrite and inhibiting the activity of extracellular signal-regulated kinase)
ANone: [] Yes, research indicates that SAC can mitigate cadmium (Cd2+) stress in rice seedlings. It achieves this by influencing the expression of genes encoding cadmium transporter proteins. SAC treatment leads to reduced expression of OsNramp5 and OsHMA2, genes involved in Cd2+ uptake and transport, while increasing OsHMA3 expression, a gene associated with vacuolar sequestration of Cd2+. This altered gene expression profile contributes to reduced Cd2+ accumulation in the roots and shoots of rice seedlings. (Ref: [Mechanism of S-allyl-L-cysteine Alleviating Cadmium Stress in Seedling Roots and Buds of Rice Seedlings])
ANone: [] Yes, encapsulating SAC within chitosan nanoparticles (SC CS NPs) has been shown to improve its brain bioavailability. Intranasal administration of SC CS NPs resulted in significant enhancements in pharmacokinetic parameters, including area under the curve (AUC) and maximum concentration (Cmax), compared to intravenous and intranasal administration of free SAC. This improved bioavailability was correlated with enhanced neuroprotective effects in an ischemic stroke rat model, as evidenced by biochemical, neurobehavioral, and histopathological analyses. (Ref: Preparation of novel S-allyl cysteine chitosan based nanoparticles for use in ischemic brain treatment)
ANone: [] Yes, S-allyl-mercapturic acid (ALMA), a urinary metabolite of SAC, can be used as a biomarker to monitor garlic consumption. ALMA is formed through the metabolic pathway of γ-glutamyl-S-allyl-L-cysteine, a precursor found in garlic. Measurement of ALMA in urine samples using techniques like gas chromatography-mass spectrometry (GC-MS) can provide insights into garlic intake and compliance in dietary intervention studies. (Ref: Biomonitoring the intake of garlic via urinary excretion of allyl mercapturic acid)
ANone: [] A variety of analytical methods have been developed and validated for the accurate quantification of SAC in various matrices. These methods include:
体外研究产品的免责声明和信息
请注意,BenchChem 上展示的所有文章和产品信息仅供信息参考。 BenchChem 上可购买的产品专为体外研究设计,这些研究在生物体外进行。体外研究,源自拉丁语 "in glass",涉及在受控实验室环境中使用细胞或组织进行的实验。重要的是要注意,这些产品没有被归类为药物或药品,他们没有得到 FDA 的批准,用于预防、治疗或治愈任何医疗状况、疾病或疾病。我们必须强调,将这些产品以任何形式引入人类或动物的身体都是法律严格禁止的。遵守这些指南对确保研究和实验的法律和道德标准的符合性至关重要。