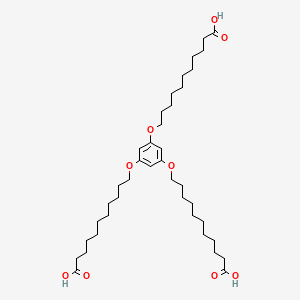
1,3,5-Tris(10-carboxydecyloxy)benzene
描述
1,3,5-Tris(10-carboxydecyloxy)benzene is a compound with the molecular formula C39H66O9. It is known for its unique structure, which consists of a benzene ring substituted with three decyloxy chains, each terminating in a carboxylic acid group. This compound is often used in the formation of self-assembled monolayers and has applications in various fields, including nanotechnology and materials science .
准备方法
The synthesis of 1,3,5-Tris(10-carboxydecyloxy)benzene typically involves the reaction of 1,3,5-trihydroxybenzene with 10-bromodecanoic acid under basic conditions. The reaction proceeds through a nucleophilic substitution mechanism, where the hydroxyl groups of the benzene ring are replaced by decyloxy chains. The reaction conditions often include the use of a base such as potassium carbonate and a solvent like dimethylformamide (DMF). The product is then purified through recrystallization or chromatography .
化学反应分析
1,3,5-Tris(10-carboxydecyloxy)benzene can undergo various chemical reactions, including:
Oxidation: The carboxylic acid groups can be oxidized to form corresponding carboxylate salts under basic conditions.
Reduction: The carboxylic acid groups can be reduced to alcohols using reducing agents such as lithium aluminum hydride (LiAlH4).
Substitution: The decyloxy chains can be substituted with other functional groups through nucleophilic substitution reactions. Common reagents used in these reactions include oxidizing agents like potassium permanganate (KMnO4) and reducing agents like LiAlH4. .
科学研究应用
Molecular Electronics
Self-Assembly and Nanostructures
TCDB has been utilized in the self-assembly of heterobilayer nanostructures. In a study involving the co-assembly of TCDB with a tetrathiafulvalene (TTF) derivative, researchers observed the formation of a hexagonal network with large nanopores. Scanning Tunneling Microscopy (STM) revealed that this network could evolve into more stable linear structures under specific conditions, indicating potential applications in molecular electronics and nanoscale device fabrication .
Template for Functionalization
The compound serves as an effective template for immobilizing other organic molecules, such as zinc phthalocyanine (Zn-Pc). The intermolecular hydrogen-bonded networks formed by TCDB allow for precise control over the spatial arrangement of guest molecules. This capability is crucial for developing advanced materials in electronic applications .
Supramolecular Chemistry
Nano-Reactor Applications
TCDB's ability to form well-defined two-dimensional networks makes it suitable for use as a nano-reactor. In experiments, TCDB facilitated the supramolecular coordination of various organic compounds, enhancing the adsorption efficiency of guest molecules due to its flexible alkyl chains and hydrogen bonding capabilities. This property is particularly valuable in synthesizing complex molecular architectures and studying reaction mechanisms at the nanoscale .
Nanotechnology
Controlled Topography for Protein Crystallization
In nanotechnology, TCDB has been employed to create surfaces with controllable topography and chemistry for protein crystallization. Researchers investigated different deposition methods (sublimation, drop casting, and dip-coating) to optimize surface properties. The results indicated that surfaces treated with TCDB could significantly influence the crystallization behavior of model proteins such as lysozyme and insulin. Hydrophilic surfaces promoted nucleation while hydrophobic surfaces yielded smaller crystals .
Deposition Method | Surface Property | Protein Response |
---|---|---|
Sublimation | Hydrophilic | Increased nucleation rates |
Drop Casting | Macrosteps | Fewer crystals formed |
Dip-Coating | Controlled roughness | Varied crystal sizes based on surface type |
Case Studies
- Nanostructure Formation : A study demonstrated that TCDB could form stable monolayers on highly oriented pyrolytic graphite (HOPG), leading to significant advances in understanding molecular packing patterns at interfaces and their implications for device design .
- Protein Crystallization : Research into protein crystallization on TCDB-modified surfaces revealed that varying exposure times affected hydrophilicity and surface roughness, ultimately influencing crystal growth outcomes. Insulin crystallized more effectively on hydrophobic surfaces with macrosteps compared to smoother ones .
作用机制
The mechanism by which 1,3,5-Tris(10-carboxydecyloxy)benzene exerts its effects is primarily through its ability to form self-assembled monolayers. These monolayers can influence the properties of surfaces, such as hydrophobicity and topography, which in turn affect the behavior of molecules interacting with the surface. The molecular targets and pathways involved include the interaction of the carboxylic acid groups with metal ions and the formation of hydrogen bonds with other molecules .
相似化合物的比较
1,3,5-Tris(10-carboxydecyloxy)benzene can be compared with other similar compounds, such as benzene-1,3,5-tricarboxamide and benzene-1,3,5-tris(10-carboxydecyloxy)benzene derivatives. These compounds share a similar benzene core structure but differ in the nature and length of the substituent chains. The uniqueness of this compound lies in its ability to form stable monolayers with specific surface properties, making it particularly useful for applications in nanotechnology and materials science .
生物活性
1,3,5-Tris(10-carboxydecyloxy)benzene (TCDB) is a compound that has garnered attention in various fields of research due to its unique structural properties and biological activity. This article explores the biological activity of TCDB, focusing on its role in protein crystallization, supramolecular coordination, and potential applications in biotechnology.
Structural Characteristics
TCDB is a triple-armed amphiphilic molecule that exhibits significant versatility in forming supramolecular structures. Its structure allows for the formation of hydrogen bonds and coordination complexes, making it a valuable template in nanotechnology and biochemistry.
Protein Crystallization
One of the most notable applications of TCDB is in enhancing protein crystallization. Research has shown that TCDB-modified surfaces significantly improve the crystallization process of various proteins. For instance, studies utilizing trypsin from bovine pancreas demonstrated that TCDB-modified Nafion membranes facilitated better nucleation and growth rates compared to unmodified surfaces .
Table 1: Effect of TCDB on Protein Crystallization
Supramolecular Coordination
TCDB has also been employed as a template for supramolecular coordination processes. In one study, its intermolecular hydrogen-bonded network was used to facilitate the coordination of zinc(II) phthalocyanine with other compounds. This process was analyzed using scanning tunneling microscopy (STM), revealing the importance of TCDB in organizing molecular interactions at the nanoscale .
Case Study 1: Enhanced Protein Crystallization
In an experiment involving TCDB-coated surfaces, researchers observed a marked improvement in protein crystallization efficiency. The study utilized various proteins including trypsin and insulin, demonstrating that the surface chemistry influenced the crystallization outcome significantly. Specifically, hydrophilic surfaces yielded fewer crystals for some proteins but enhanced nucleation for others like insulin .
Case Study 2: Supramolecular Assemblies
Another study focused on the use of TCDB in creating two-dimensional host networks for molecular assemblies. The research highlighted how TCDB could organize other molecules effectively through hydrogen bonding, thus impacting their biological function and interaction with target substrates .
常见问题
Basic Research Questions
Q. What are the optimal synthetic routes for 1,3,5-Tris(10-carboxydecyloxy)benzene, and how can reaction conditions be systematically optimized?
- Methodological Answer : The synthesis typically involves nucleophilic substitution or esterification reactions under reflux conditions. For example, analogous triazole derivatives are synthesized by refluxing precursors in absolute ethanol with catalytic glacial acetic acid, followed by solvent evaporation and purification via recrystallization . Systematic optimization can employ fractional factorial design to test variables (e.g., temperature, molar ratios, catalyst concentration) and identify critical parameters. Reaction progress should be monitored using thin-layer chromatography (TLC) to determine endpoint efficiency.
Q. Which spectroscopic and chromatographic techniques are most effective for characterizing the purity and structural integrity of this compound?
- Methodological Answer : Nuclear Magnetic Resonance (NMR) spectroscopy (¹H, ¹³C) resolves the aromatic core and alkyl chain configurations, while Fourier-Transform Infrared (FT-IR) spectroscopy confirms ester and carboxylic acid functional groups. High-Performance Liquid Chromatography (HPLC) with UV detection quantifies purity, and Mass Spectrometry (MS) validates molecular weight. For structural elucidation of byproducts, tandem MS/MS or 2D NMR (e.g., COSY, HSQC) is recommended .
Q. What strategies mitigate ester group hydrolysis during synthesis and storage of this compound?
- Methodological Answer : Hydrolysis can be minimized by maintaining anhydrous conditions using molecular sieves or inert atmospheres (N₂/Ar). During synthesis, avoid aqueous workup unless necessary. For storage, lyophilize the compound and store at -20°C under desiccation. Buffered solutions (pH 4–6) reduce carboxylate ionization, stabilizing the ester linkages .
Q. How can researchers validate synthetic reproducibility across different laboratories?
- Methodological Answer : Implement a standardized protocol with detailed reaction parameters (e.g., stirring rate, solvent grade, drying methods). Use a pre-test/post-test design with a shared batch of starting materials to compare yields and purity metrics (e.g., HPLC area%) across labs. Statistical tools like interlaboratory coefficient of variation (CV) analysis identify procedural inconsistencies .
Advanced Research Questions
Q. How can computational modeling predict the self-assembly behavior of this compound in supramolecular systems?
- Methodological Answer : Molecular dynamics (MD) simulations with force fields (e.g., CHARMM, AMBER) model the compound’s aggregation in solvent environments. Parameters include solvation energy, π-π stacking interactions, and carboxylate group orientation. COMSOL Multiphysics® integrates these models with experimental data to predict micelle or vesicle formation . Validate predictions using Small-Angle X-ray Scattering (SAXS) and cryogenic electron microscopy (cryo-EM).
Q. What methodological considerations are critical when investigating the compound’s potential as a drug delivery vehicle?
- Methodological Answer : Focus on encapsulation efficiency (e.g., using fluorescent probes) and pH-responsive release profiles. In vitro assays should simulate physiological conditions (e.g., PBS at pH 7.4 vs. lysosomal pH 5.0). Use dynamic light scattering (DLS) to monitor nanoparticle stability and cytotoxicity assays (MTT/CCK-8) on cell lines. Theoretical frameworks linking molecular structure to payload release kinetics guide experimental design .
Q. How do factorial design approaches enhance the efficiency of structure-property relationship studies?
- Methodological Answer : A 2³ factorial design evaluates three variables (e.g., alkyl chain length, carboxylate density, solvent polarity) across eight experimental conditions. Response surface methodology (RSM) identifies nonlinear interactions, optimizing properties like critical micelle concentration (CMC) or solubility. Statistical software (e.g., Minitab, JMP) analyzes significance (p < 0.05) and generates predictive models .
Q. What advanced separation techniques are required to isolate stereoisomers or byproducts of this compound?
- Methodological Answer : Chiral HPLC with polysaccharide-based columns (e.g., Chiralpak®) resolves stereoisomers. For byproducts, use preparative-scale HPLC with gradient elution or membrane-based separation technologies (e.g., ultrafiltration, dialysis) to isolate high-purity fractions. Mass-directed purification systems (e.g., LC-MS) streamline identification .
Q. How can researchers integrate experimental data with theoretical frameworks in supramolecular chemistry studies?
- Methodological Answer : Apply density functional theory (DFT) to calculate electronic properties (e.g., HOMO-LUMO gaps) and correlate with experimental UV-Vis spectra. Use Scherrer analysis of XRD data to validate crystal structure predictions. Theoretical frameworks from soft matter physics (e.g., Flory-Huggins theory) explain self-assembly thermodynamics .
Q. What multi-scale modeling approaches are used to study the compound’s interfacial behavior in composite materials?
- Methodological Answer : Coarse-grained MD simulations model large-scale interactions at polymer interfaces, while finite element analysis (FEA) predicts mechanical properties (e.g., tensile strength). Machine learning algorithms trained on experimental datasets optimize surfactant or crosslinking agent ratios. Validate with atomic force microscopy (AFM) and interfacial rheometry .
属性
IUPAC Name |
11-[3,5-bis(10-carboxydecoxy)phenoxy]undecanoic acid | |
---|---|---|
Details | Computed by Lexichem TK 2.7.0 (PubChem release 2021.05.07) | |
Source | PubChem | |
URL | https://pubchem.ncbi.nlm.nih.gov | |
Description | Data deposited in or computed by PubChem | |
InChI |
InChI=1S/C39H66O9/c40-37(41)25-19-13-7-1-4-10-16-22-28-46-34-31-35(47-29-23-17-11-5-2-8-14-20-26-38(42)43)33-36(32-34)48-30-24-18-12-6-3-9-15-21-27-39(44)45/h31-33H,1-30H2,(H,40,41)(H,42,43)(H,44,45) | |
Details | Computed by InChI 1.0.6 (PubChem release 2021.05.07) | |
Source | PubChem | |
URL | https://pubchem.ncbi.nlm.nih.gov | |
Description | Data deposited in or computed by PubChem | |
InChI Key |
MCFBTTOOZOQCNF-UHFFFAOYSA-N | |
Details | Computed by InChI 1.0.6 (PubChem release 2021.05.07) | |
Source | PubChem | |
URL | https://pubchem.ncbi.nlm.nih.gov | |
Description | Data deposited in or computed by PubChem | |
Canonical SMILES |
C1=C(C=C(C=C1OCCCCCCCCCCC(=O)O)OCCCCCCCCCCC(=O)O)OCCCCCCCCCCC(=O)O | |
Details | Computed by OEChem 2.3.0 (PubChem release 2021.05.07) | |
Source | PubChem | |
URL | https://pubchem.ncbi.nlm.nih.gov | |
Description | Data deposited in or computed by PubChem | |
Molecular Formula |
C39H66O9 | |
Details | Computed by PubChem 2.1 (PubChem release 2021.05.07) | |
Source | PubChem | |
URL | https://pubchem.ncbi.nlm.nih.gov | |
Description | Data deposited in or computed by PubChem | |
DSSTOX Substance ID |
DTXSID80758077 | |
Record name | 11,11',11''-[Benzene-1,3,5-triyltris(oxy)]triundecanoic acid | |
Source | EPA DSSTox | |
URL | https://comptox.epa.gov/dashboard/DTXSID80758077 | |
Description | DSSTox provides a high quality public chemistry resource for supporting improved predictive toxicology. | |
Molecular Weight |
678.9 g/mol | |
Details | Computed by PubChem 2.1 (PubChem release 2021.05.07) | |
Source | PubChem | |
URL | https://pubchem.ncbi.nlm.nih.gov | |
Description | Data deposited in or computed by PubChem | |
CAS No. |
92631-02-2 | |
Record name | 11,11',11''-[Benzene-1,3,5-triyltris(oxy)]triundecanoic acid | |
Source | EPA DSSTox | |
URL | https://comptox.epa.gov/dashboard/DTXSID80758077 | |
Description | DSSTox provides a high quality public chemistry resource for supporting improved predictive toxicology. | |
Retrosynthesis Analysis
AI-Powered Synthesis Planning: Our tool employs the Template_relevance Pistachio, Template_relevance Bkms_metabolic, Template_relevance Pistachio_ringbreaker, Template_relevance Reaxys, Template_relevance Reaxys_biocatalysis model, leveraging a vast database of chemical reactions to predict feasible synthetic routes.
One-Step Synthesis Focus: Specifically designed for one-step synthesis, it provides concise and direct routes for your target compounds, streamlining the synthesis process.
Accurate Predictions: Utilizing the extensive PISTACHIO, BKMS_METABOLIC, PISTACHIO_RINGBREAKER, REAXYS, REAXYS_BIOCATALYSIS database, our tool offers high-accuracy predictions, reflecting the latest in chemical research and data.
Strategy Settings
Precursor scoring | Relevance Heuristic |
---|---|
Min. plausibility | 0.01 |
Model | Template_relevance |
Template Set | Pistachio/Bkms_metabolic/Pistachio_ringbreaker/Reaxys/Reaxys_biocatalysis |
Top-N result to add to graph | 6 |
Feasible Synthetic Routes
体外研究产品的免责声明和信息
请注意,BenchChem 上展示的所有文章和产品信息仅供信息参考。 BenchChem 上可购买的产品专为体外研究设计,这些研究在生物体外进行。体外研究,源自拉丁语 "in glass",涉及在受控实验室环境中使用细胞或组织进行的实验。重要的是要注意,这些产品没有被归类为药物或药品,他们没有得到 FDA 的批准,用于预防、治疗或治愈任何医疗状况、疾病或疾病。我们必须强调,将这些产品以任何形式引入人类或动物的身体都是法律严格禁止的。遵守这些指南对确保研究和实验的法律和道德标准的符合性至关重要。