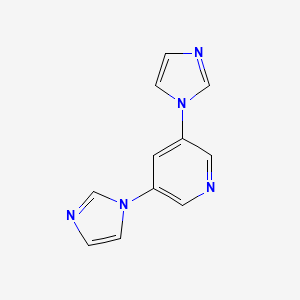
3,5-二(1H-咪唑-1-基)吡啶
描述
3,5-Di(1h-imidazol-1-yl)pyridine is a compound that contains a pyridine ring substituted with two imidazole groups at the 3rd and 5th positions . The N-donor ligand 3,5-bis (1-imidazoly)pyridine (bip) is introduced as a secondary ligand to modify the structure of the resulting compound .
Synthesis Analysis
The solvothermal synthesis of three new 2D MOFs is reported. The reaction of 3,5-di(1H-imidazol-1-yl)pyridine (dip), and the metal salts Co(NO3)2·6H2O, Cd(NO3)2·4H2O and Zn(NO3)2·4H2O, resulted in the formation of three MOFs [Co(dip)2(SCN)2] (1), {[Cd(dip)2(H2O)2]·(NO3)2} (2), {[Zn(dip)2(H2O)2]·(ClO4)2} (3) .Molecular Structure Analysis
For these 2D MOFs, all the metal ions adopted six-coordinated octahedral geometry with axial coordination sites occupied by SCN- or water molecules . The asymmetric unit comprises of one half Mn (II) cation, one Hcbaa anion, one bip molecule and one coordinated H2O molecule .Chemical Reactions Analysis
Imidazole is a five-membered heterocyclic moiety that possesses three carbon, two nitrogen, four hydrogen atoms, and two double bonds . It shows both acidic and basic properties and is highly soluble in water and other polar solvents . Due to the presence of a positive charge on either of two nitrogen atoms, it shows two equivalent tautomeric forms .Physical And Chemical Properties Analysis
Imidazole is a white or colorless solid that is highly soluble in water and other polar solvents . It is amphoteric in nature, meaning it shows both acidic and basic properties .科学研究应用
Synthesis of 2D MOFs: The solvothermal synthesis of three new 2D MOFs involving dip and metal salts (Co(NO3)2·6H2O, Cd(NO3)2·4H2O, and Zn(NO3)2·4H2O) resulted in the following compounds:
In these 2D MOFs, all metal ions adopt six-coordinated octahedral geometry, with axial coordination sites occupied by SCN- or water molecules. The monocoordinated ligands contribute to the layered MOF structure .
Fluorescence Properties: The fluorescence properties of compounds (2) and (3) have been investigated. Understanding their luminescent behavior is crucial for potential applications in sensors, imaging, and optoelectronics.
Anti-Liver Cancer Activity Evaluation
While researching dip, scientists have explored its potential as an anti-liver cancer agent. Crystal structures and biological evaluations have been conducted to assess its efficacy . Further studies are needed to elucidate the underlying mechanisms and optimize its therapeutic potential.
未来方向
The development of new drugs that overcome the AMR problems is necessary . In the past, those drugs which contain heterocyclic nuclei give high chemotherapeutic values and act as a remedy for the development of novel drugs . There are lots of heterocyclic compounds that are in clinical use to treat infectious diseases . Therefore, there is a great importance of heterocyclic ring containing drugs .
作用机制
Target of Action
The primary targets of 3,5-Di(1h-imidazol-1-yl)pyridine are metal ions, specifically Co , Cd , and Zn . These metal ions play a crucial role in the formation of two-dimensional metal-organic frameworks (2D MOFs), which are the primary structures formed by this compound .
Mode of Action
3,5-Di(1h-imidazol-1-yl)pyridine interacts with its targets by forming coordination polymers. In these structures, all the metal ions adopt a six-coordinated octahedral geometry with axial coordination sites occupied by SCN- or water molecules . This interaction leads to the formation of layered MOF structures .
Biochemical Pathways
The formation of 2d mofs suggests potential applications in areas such as gas storage, catalysis, energy storage, luminescent materials, and solar energy utilization .
Result of Action
The primary result of the action of 3,5-Di(1h-imidazol-1-yl)pyridine is the formation of 2D MOFs . These structures have potential applications in various fields due to their tailorable structure, porous character, and large surface area . Furthermore, the fluorescence properties of some of these MOFs have been investigated .
Action Environment
The action of 3,5-Di(1h-imidazol-1-yl)pyridine is influenced by environmental factors such as the presence of metal ions and the conditions under which the reaction takes place . For instance, the solvothermal synthesis method is used to create the 2D MOFs
属性
IUPAC Name |
3,5-di(imidazol-1-yl)pyridine | |
---|---|---|
Details | Computed by Lexichem TK 2.7.0 (PubChem release 2021.05.07) | |
Source | PubChem | |
URL | https://pubchem.ncbi.nlm.nih.gov | |
Description | Data deposited in or computed by PubChem | |
InChI |
InChI=1S/C11H9N5/c1-3-15(8-12-1)10-5-11(7-14-6-10)16-4-2-13-9-16/h1-9H | |
Details | Computed by InChI 1.0.6 (PubChem release 2021.05.07) | |
Source | PubChem | |
URL | https://pubchem.ncbi.nlm.nih.gov | |
Description | Data deposited in or computed by PubChem | |
InChI Key |
FBPFYCGCNHLTGV-UHFFFAOYSA-N | |
Details | Computed by InChI 1.0.6 (PubChem release 2021.05.07) | |
Source | PubChem | |
URL | https://pubchem.ncbi.nlm.nih.gov | |
Description | Data deposited in or computed by PubChem | |
Canonical SMILES |
C1=CN(C=N1)C2=CC(=CN=C2)N3C=CN=C3 | |
Details | Computed by OEChem 2.3.0 (PubChem release 2021.05.07) | |
Source | PubChem | |
URL | https://pubchem.ncbi.nlm.nih.gov | |
Description | Data deposited in or computed by PubChem | |
Molecular Formula |
C11H9N5 | |
Details | Computed by PubChem 2.1 (PubChem release 2021.05.07) | |
Source | PubChem | |
URL | https://pubchem.ncbi.nlm.nih.gov | |
Description | Data deposited in or computed by PubChem | |
Molecular Weight |
211.22 g/mol | |
Details | Computed by PubChem 2.1 (PubChem release 2021.05.07) | |
Source | PubChem | |
URL | https://pubchem.ncbi.nlm.nih.gov | |
Description | Data deposited in or computed by PubChem | |
Product Name |
3,5-Di(1h-imidazol-1-yl)pyridine |
Retrosynthesis Analysis
AI-Powered Synthesis Planning: Our tool employs the Template_relevance Pistachio, Template_relevance Bkms_metabolic, Template_relevance Pistachio_ringbreaker, Template_relevance Reaxys, Template_relevance Reaxys_biocatalysis model, leveraging a vast database of chemical reactions to predict feasible synthetic routes.
One-Step Synthesis Focus: Specifically designed for one-step synthesis, it provides concise and direct routes for your target compounds, streamlining the synthesis process.
Accurate Predictions: Utilizing the extensive PISTACHIO, BKMS_METABOLIC, PISTACHIO_RINGBREAKER, REAXYS, REAXYS_BIOCATALYSIS database, our tool offers high-accuracy predictions, reflecting the latest in chemical research and data.
Strategy Settings
Precursor scoring | Relevance Heuristic |
---|---|
Min. plausibility | 0.01 |
Model | Template_relevance |
Template Set | Pistachio/Bkms_metabolic/Pistachio_ringbreaker/Reaxys/Reaxys_biocatalysis |
Top-N result to add to graph | 6 |
Feasible Synthetic Routes
体外研究产品的免责声明和信息
请注意,BenchChem 上展示的所有文章和产品信息仅供信息参考。 BenchChem 上可购买的产品专为体外研究设计,这些研究在生物体外进行。体外研究,源自拉丁语 "in glass",涉及在受控实验室环境中使用细胞或组织进行的实验。重要的是要注意,这些产品没有被归类为药物或药品,他们没有得到 FDA 的批准,用于预防、治疗或治愈任何医疗状况、疾病或疾病。我们必须强调,将这些产品以任何形式引入人类或动物的身体都是法律严格禁止的。遵守这些指南对确保研究和实验的法律和道德标准的符合性至关重要。