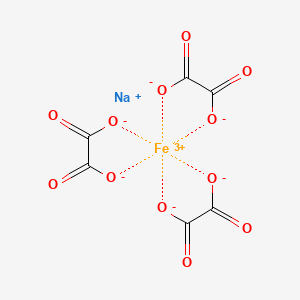
草酸铁钠
描述
Ferric Sodium Oxalate is an emerald-green, crystalline, extremely water-soluble salt . It is used in photography and blueprinting .
Synthesis Analysis
Ferric Sodium Oxalate can be synthesized by mixing solutions of sodium oxalate and ferric oxalate . The reaction takes a few hours for the brown color of the ferric oxalate to be replaced with the green color of the complex anion . The balanced chemical reaction equation for the formation of the potassium ferrioxalate salt is:FeCl3•6H2O (aq) + 3K2C2O4•H2O (aq) → K3Fe (C2O4)3•3H2O (s) + 3KCl (aq) + 6H2O (l)
. Molecular Structure Analysis
The compound is a salt consisting of ferrioxalate anions, [Fe(C2O4)3]3−, and sodium cations Na+ . The anion is a transition metal complex consisting of an iron atom in the +3 oxidation state and three bidentate oxalate ions C2O2−4 anions serving as ligands . The bonds to the iron atom are dative covalent bonds .Chemical Reactions Analysis
The ferrioxalate anion is sensitive to light and higher-energy electromagnetic radiation, which causes the decomposition of one oxalate to carbon dioxide (CO2) and reduction of the iron (III) atom to iron (II) .Physical And Chemical Properties Analysis
Ferric Sodium Oxalate is an emerald-green, crystalline, extremely water-soluble salt . It has a molar mass of 388.868 g·mol−1 . It appears as lime green hydrated crystals . It has a density of 1.97 g/cm3 at 17 °C . It is soluble in water .科学研究应用
Fenton Catalytic Performance
Ferrous oxalate dihydrate (FOD), which can be synthesized from ferric oxalate, is used as a photo-Fenton catalyst with remarkable catalytic and photocatalytic performances on organic pollutant degradation . It has been used in the degradation of methylene blue, a common dye pollutant .
Photolysis Research
Ferric oxalate is used in photolysis research, specifically in studying the mechanisms of iron carboxylate photolysis reactions . The photolysis of ferric oxalate and the eventual production of CO2 is a topic of ongoing research .
Environmental Science
In environmental science, ferric oxalate is used to quantify the flux of light in aqueous systems . It serves as a model complex for understanding metal carboxylate complex photolysis .
Photographic Printing Process
Ferric oxalate is used as the light-sensitive element in the Kallitype photographic printing process . It’s also used in the platinotype process of Platinum/Palladium Printing .
Battery Technology
Ferric oxalate tetrahydrate has been investigated as a possible cheap material for the positive electrode of lithium-iron batteries .
Wastewater Treatment
Ferrous oxalate dihydrate (FOD) synthesized from ferric oxalate has been used in wastewater treatment. It has shown high photo-Fenton degradation efficiency and reduced reaction time for wastewater treatment .
作用机制
Target of Action
Ferric sodium oxalate, also known as sodium ferrioxalate, is a complex consisting of an iron atom in the +3 oxidation state and three bidentate oxalate ions . The primary targets of ferric sodium oxalate are the iron (III) atoms and the oxalate ions present in the compound .
Mode of Action
The mode of action of ferric sodium oxalate involves a process known as photoreduction . This process is initiated when the ferrioxalate anion absorbs light or higher-energy electromagnetic radiation . This absorption triggers an intramolecular electron transfer from the oxalate to the iron, which occurs on a sub-picosecond timescale, creating an iron (II) complexed by one oxidized and two spectator oxalate ligands .
Biochemical Pathways
The biochemical pathway affected by ferric sodium oxalate is the photolysis reaction pathway . Following the electron transfer, the oxidized oxalate molecule dissociates to form free solvated carbon dioxide (CO2) and a species inferred to be CO2^- based on the appearance of a new vibrational absorption band . This process is part of the iron redox cycle and is a major pathway for the mineralization of dissolved organic matter in sunlit waters and atmospheric aerosol particles .
Pharmacokinetics
It’s known that the compound is slightly soluble in water , which may influence its bioavailability.
Result of Action
The result of the action of ferric sodium oxalate is the reduction of the iron (III) atom to iron (II) and the decomposition of one oxalate to carbon dioxide (CO2) . This process results in the formation of an iron (II) complex and free solvated CO2 .
Action Environment
The action of ferric sodium oxalate is influenced by environmental factors such as light and higher-energy electromagnetic radiation . The compound is sensitive to these factors, which trigger the photoreduction process . Additionally, the reaction equilibrium is attained only slowly at room temperature .
安全和危害
Ferric Sodium Oxalate is corrosive and can cause eye, respiratory, and skin irritation . It is recommended to wear personal protective equipment/face protection, ensure adequate ventilation, avoid dust formation, avoid contact with skin, eyes, or clothing, avoid ingestion and inhalation, and keep containers tightly closed in a dry, cool, and well-ventilated place .
属性
IUPAC Name |
sodium;iron(3+);oxalate | |
---|---|---|
Details | Computed by Lexichem TK 2.7.0 (PubChem release 2021.08.13) | |
Source | PubChem | |
URL | https://pubchem.ncbi.nlm.nih.gov | |
Description | Data deposited in or computed by PubChem | |
InChI |
InChI=1S/3C2H2O4.Fe.Na/c3*3-1(4)2(5)6;;/h3*(H,3,4)(H,5,6);;/q;;;+3;+1/p-6 | |
Details | Computed by InChI 1.0.6 (PubChem release 2021.08.13) | |
Source | PubChem | |
URL | https://pubchem.ncbi.nlm.nih.gov | |
Description | Data deposited in or computed by PubChem | |
InChI Key |
WIHZODBNOVPFSA-UHFFFAOYSA-H | |
Details | Computed by InChI 1.0.6 (PubChem release 2021.08.13) | |
Source | PubChem | |
URL | https://pubchem.ncbi.nlm.nih.gov | |
Description | Data deposited in or computed by PubChem | |
Canonical SMILES |
C(=O)(C(=O)[O-])[O-].C(=O)(C(=O)[O-])[O-].C(=O)(C(=O)[O-])[O-].[Na+].[Fe+3] | |
Details | Computed by OEChem 2.3.0 (PubChem release 2021.08.13) | |
Source | PubChem | |
URL | https://pubchem.ncbi.nlm.nih.gov | |
Description | Data deposited in or computed by PubChem | |
Molecular Formula |
C6FeNaO12-2 | |
Details | Computed by PubChem 2.1 (PubChem release 2021.08.13) | |
Source | PubChem | |
URL | https://pubchem.ncbi.nlm.nih.gov | |
Description | Data deposited in or computed by PubChem | |
Molecular Weight |
342.89 g/mol | |
Details | Computed by PubChem 2.1 (PubChem release 2021.08.13) | |
Source | PubChem | |
URL | https://pubchem.ncbi.nlm.nih.gov | |
Description | Data deposited in or computed by PubChem | |
CAS RN |
5936-14-1 | |
Record name | Ferrate(3-), tris[ethanedioato(2-)-κO1,κO2]-, sodium (1:3) | |
Source | CAS Common Chemistry | |
URL | https://commonchemistry.cas.org/detail?cas_rn=5936-14-1 | |
Description | CAS Common Chemistry is an open community resource for accessing chemical information. Nearly 500,000 chemical substances from CAS REGISTRY cover areas of community interest, including common and frequently regulated chemicals, and those relevant to high school and undergraduate chemistry classes. This chemical information, curated by our expert scientists, is provided in alignment with our mission as a division of the American Chemical Society. | |
Explanation | The data from CAS Common Chemistry is provided under a CC-BY-NC 4.0 license, unless otherwise stated. | |
Retrosynthesis Analysis
AI-Powered Synthesis Planning: Our tool employs the Template_relevance Pistachio, Template_relevance Bkms_metabolic, Template_relevance Pistachio_ringbreaker, Template_relevance Reaxys, Template_relevance Reaxys_biocatalysis model, leveraging a vast database of chemical reactions to predict feasible synthetic routes.
One-Step Synthesis Focus: Specifically designed for one-step synthesis, it provides concise and direct routes for your target compounds, streamlining the synthesis process.
Accurate Predictions: Utilizing the extensive PISTACHIO, BKMS_METABOLIC, PISTACHIO_RINGBREAKER, REAXYS, REAXYS_BIOCATALYSIS database, our tool offers high-accuracy predictions, reflecting the latest in chemical research and data.
Strategy Settings
Precursor scoring | Relevance Heuristic |
---|---|
Min. plausibility | 0.01 |
Model | Template_relevance |
Template Set | Pistachio/Bkms_metabolic/Pistachio_ringbreaker/Reaxys/Reaxys_biocatalysis |
Top-N result to add to graph | 6 |
Feasible Synthetic Routes
体外研究产品的免责声明和信息
请注意,BenchChem 上展示的所有文章和产品信息仅供信息参考。 BenchChem 上可购买的产品专为体外研究设计,这些研究在生物体外进行。体外研究,源自拉丁语 "in glass",涉及在受控实验室环境中使用细胞或组织进行的实验。重要的是要注意,这些产品没有被归类为药物或药品,他们没有得到 FDA 的批准,用于预防、治疗或治愈任何医疗状况、疾病或疾病。我们必须强调,将这些产品以任何形式引入人类或动物的身体都是法律严格禁止的。遵守这些指南对确保研究和实验的法律和道德标准的符合性至关重要。