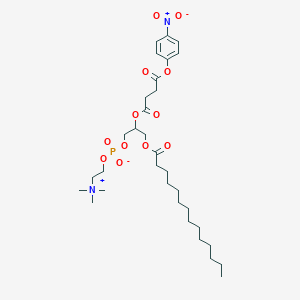
sn-甘油-3-磷酸胆碱 A
描述
Sn-Glycero-3-phosphocholine, also known as Choline Alfoscerate, is a nootropic phospholipid. It is a precursor to choline biosynthesis and an intermediate in the metabolism of phosphatidylcholine . It increases the bioavailability of choline in nervous tissue and has significant effects on cognitive function .
Synthesis Analysis
Sn-Glycero-3-phosphocholine is a primary component of biological membranes and plays a critical role in membrane dynamics and structure . It is synthesized by two distinct pathways. The de novo pathway utilizes 1-alkyl-2-acetyl-sn-glycerol and CDP-choline as substrates of a DTT-insensitive phosphocholine transferase (PAF-PCT). The remodeling pathway requires the production of 1-alkyl-2-lyso-sn-glycero-3-phosphocholine (lysoPAF) produced by the hydrolysis of 1-alkyl-2-(long-chain)acyl-sn-glycero-3-phosphocholine (alkylacylGPC) by the action of phospholipases A2 .Molecular Structure Analysis
The molecular formula of sn-Glycero-3-phosphocholine is C8H20NO6P . It has a molecular weight of 257.2 .Chemical Reactions Analysis
In vivo, sn-Glycero-3-phosphocholine is converted to phosphorylcholine, a metabolically active form of choline that increases acetylcholine synthesis and release in the rat hippocampus . It also participates in the metabolism of phosphatidylcholine .Physical And Chemical Properties Analysis
Sn-Glycero-3-phosphocholine is a white powder . It has a specific rotation of -2.7° and a pH of 7.5 .科学研究应用
Neurotherapy and Drug Delivery
sn-Glycero-3-phosphocholine plays a crucial role in neurotherapy and drug delivery. Researchers have explored its potential in enhancing drug transport across the blood-brain barrier. Specifically, 1,2-Dimyristoyl-sn-glycero-3-phosphocholine (DMPC) coated on the surface of superparamagnetic iron oxide nanoparticles (SPIONs) has shown promise . These modified nanoparticles effectively adhere to bio-membranes and can be used for targeted drug delivery to brain tissues. DMPC’s similarity to brain cell membranes facilitates its attachment to axons, dendrites, and myelin sheaths, making it a valuable candidate for drug delivery in neurological disorders.
Choline Precursor for Acetylcholine Synthesis
sn-Glycero-3-phosphocholine rapidly delivers choline to the brain across the blood-brain barrier. As a biosynthetic precursor of the acetylcholine neurotransmitter, it has potential applications in treating conditions related to cholinergic dysfunction, such as Alzheimer’s disease and dementia . By providing choline, sn-Glycero-3-phosphocholine supports acetylcholine synthesis, which is essential for cognitive function and memory.
Cognitive Enhancement
Deacylated derivatives of sn-Glycero-3-phosphocholine, such as sn-glycero-3-phosphocholine (GPC), have cognitive-enhancing properties. GPC has been studied for its ability to improve memory and cognitive performance. It may enhance neuronal membrane function and promote overall brain health . Researchers continue to explore its potential in supporting cognitive function and preventing age-related cognitive decline.
Neuroprotective Effects
sn-Glycero-3-phosphocholine exerts neuroprotective effects in models of altered cholinergic neurotransmission and brain vascular injury. It prevents scopolamine-induced amnesia in animal tests and shows promise as a natural anti-inflammatory agent . These properties make it relevant for protecting brain health and mitigating neurodegenerative processes.
作用机制
Target of Action
The primary target of sn-Glycero-3-phosphocholine A, also known as 14:0 NPS PC, is the lipoprotein-associated phospholipase A2 . This enzyme plays a crucial role in the metabolism of phosphatidylcholine, a primary component of biological membranes .
Mode of Action
The compound interacts with its target by associating with lipids or detergents, which is essential for preserving the active structure of lipoprotein-associated phospholipase A2 . This interaction leads to an increase in acetylcholine synthesis and release in the brain .
Biochemical Pathways
sn-Glycero-3-phosphocholine A is a precursor to choline biosynthesis and an intermediate in the metabolism of phosphatidylcholine . In vivo, it is converted to phosphorylcholine, a metabolically active form of choline . This conversion affects the cholinergic neurotransmission pathway, leading to increased synthesis and release of acetylcholine, a key neurotransmitter in the brain .
Pharmacokinetics
It is known that sn-glycero-3-phosphocholine a can rapidly deliver choline to the brain across the blood-brain barrier , suggesting good bioavailability.
Result of Action
The action of sn-Glycero-3-phosphocholine A leads to increased acetylcholine synthesis and release in the brain . This can enhance cognitive function and has potential implications for the treatment of conditions like Alzheimer’s disease and dementia .
Action Environment
It is soluble in ethanol, dmso, and a mixture of chloroform, methanol, and water , suggesting that its action might be influenced by the solvent environment.
安全和危害
未来方向
属性
IUPAC Name |
[2-[4-(4-nitrophenoxy)-4-oxobutanoyl]oxy-3-tetradecanoyloxypropyl] 2-(trimethylazaniumyl)ethyl phosphate | |
---|---|---|
Details | Computed by LexiChem 2.6.6 (PubChem release 2019.06.18) | |
Source | PubChem | |
URL | https://pubchem.ncbi.nlm.nih.gov | |
Description | Data deposited in or computed by PubChem | |
InChI |
InChI=1S/C32H53N2O12P/c1-5-6-7-8-9-10-11-12-13-14-15-16-30(35)42-25-29(26-44-47(40,41)43-24-23-34(2,3)4)46-32(37)22-21-31(36)45-28-19-17-27(18-20-28)33(38)39/h17-20,29H,5-16,21-26H2,1-4H3 | |
Details | Computed by InChI 1.0.5 (PubChem release 2019.06.18) | |
Source | PubChem | |
URL | https://pubchem.ncbi.nlm.nih.gov | |
Description | Data deposited in or computed by PubChem | |
InChI Key |
XOCHZRRLIIQGMZ-UHFFFAOYSA-N | |
Details | Computed by InChI 1.0.5 (PubChem release 2019.06.18) | |
Source | PubChem | |
URL | https://pubchem.ncbi.nlm.nih.gov | |
Description | Data deposited in or computed by PubChem | |
Canonical SMILES |
CCCCCCCCCCCCCC(=O)OCC(COP(=O)([O-])OCC[N+](C)(C)C)OC(=O)CCC(=O)OC1=CC=C(C=C1)[N+](=O)[O-] | |
Details | Computed by OEChem 2.1.5 (PubChem release 2019.06.18) | |
Source | PubChem | |
URL | https://pubchem.ncbi.nlm.nih.gov | |
Description | Data deposited in or computed by PubChem | |
Molecular Formula |
C32H53N2O12P | |
Details | Computed by PubChem 2.1 (PubChem release 2019.06.18) | |
Source | PubChem | |
URL | https://pubchem.ncbi.nlm.nih.gov | |
Description | Data deposited in or computed by PubChem | |
Molecular Weight |
688.7 g/mol | |
Details | Computed by PubChem 2.1 (PubChem release 2021.05.07) | |
Source | PubChem | |
URL | https://pubchem.ncbi.nlm.nih.gov | |
Description | Data deposited in or computed by PubChem | |
Product Name |
sn-Glycero-3-phosphocholine A |
Retrosynthesis Analysis
AI-Powered Synthesis Planning: Our tool employs the Template_relevance Pistachio, Template_relevance Bkms_metabolic, Template_relevance Pistachio_ringbreaker, Template_relevance Reaxys, Template_relevance Reaxys_biocatalysis model, leveraging a vast database of chemical reactions to predict feasible synthetic routes.
One-Step Synthesis Focus: Specifically designed for one-step synthesis, it provides concise and direct routes for your target compounds, streamlining the synthesis process.
Accurate Predictions: Utilizing the extensive PISTACHIO, BKMS_METABOLIC, PISTACHIO_RINGBREAKER, REAXYS, REAXYS_BIOCATALYSIS database, our tool offers high-accuracy predictions, reflecting the latest in chemical research and data.
Strategy Settings
Precursor scoring | Relevance Heuristic |
---|---|
Min. plausibility | 0.01 |
Model | Template_relevance |
Template Set | Pistachio/Bkms_metabolic/Pistachio_ringbreaker/Reaxys/Reaxys_biocatalysis |
Top-N result to add to graph | 6 |
Feasible Synthetic Routes
Q & A
Q1: What is the role of cholesterol in phospholipid membranes?
A: Cholesterol plays a crucial chemoprotective role in lipid membranes. Studies using field-induced droplet ionization mass spectrometry revealed that adding 30 mol% cholesterol to 1,2-dipalmitoyl-sn-glycero-3-phosphocholine (DPPC) or 1-palmitoyl-2-oleoyl-sn-glycero-3-phospho-(1′-rac-glycerol) (POPG) monolayers significantly reduced oxidation and ester cleavage caused by reactive species like hydroxyl radicals and HCl vapor. This protection is attributed to cholesterol's ability to increase lipid packing density and alter membrane fluidity. []
Q2: How does the presence of double bonds in polyunsaturated fatty acids affect membrane properties?
A: Double bonds in polyunsaturated fatty acids significantly impact membrane fluidity and interactions with other lipids. Research comparing membranes containing docosahexaenoic acid (DHA, 22:6n3) and docosapentaenoic acid (DPA, 22:5n6) revealed that the additional double bond in DHA increased its flexibility at the methyl end, leading to shorter isomerization correlation times. This difference in flexibility also influences the packing of saturated fatty acids within the membrane. []
Q3: How does the structure of amphipathic α-helical peptides influence their interaction with phospholipids?
A: The structure of amphipathic α-helical peptides directly impacts their ability to form discoidal phospholipid complexes called nanodiscs. Comparing nanodiscs formed by the peptide 18A and apolipoprotein A-I (apoA-I) revealed key differences. While 18A nanodiscs maintain a planar lipid bilayer irrespective of size, apoA-I nanodiscs exhibit size-dependent bilayer deformation into a saddle surface. This difference is attributed to the flexible helix chain structure of apoA-I. []
Q4: What role does acetyl-CoA:1-O-alkyl-2-lyso-sn-glycero-3-phosphocholine acetyltransferase play in inflammation?
A: Acetyl-CoA:1-O-alkyl-2-lyso-sn-glycero-3-phosphocholine acetyltransferase is a key enzyme in the biosynthesis of platelet-activating factor (PAF) via the remodeling pathway. Studies in human neutrophils demonstrated that this enzyme is directly activated by p38 kinase, a mitogen-activated protein kinase. Stimuli like tumor necrosis factor-α (TNF-α) that activate p38 kinase increased acetyltransferase activity, while inhibitors of p38 kinase abolished this activation. This suggests a critical role for p38 kinase-mediated acetyltransferase activation in inflammation. []
Q5: How do cholesterol levels affect phospholipid repair mechanisms in red blood cells?
A: Elevated cholesterol levels in red blood cell membranes inhibit phospholipid repair mechanisms. Studies show that cholesterol-loading decreases the incorporation of arachidonate into phospholipids and increases its uptake into acylcarnitine, a fatty acid repair intermediate. This inhibition is linked to decreased activity of arachidonoyl-CoA:l-palmitoyl-sn-glycero-3-phosphocholine acyl transferase (LAT), a key enzyme in the phospholipid repair pathway. []
Q6: Is there a difference in the synthesis of PAF and 1-acyl-2-acetyl-sn-glycero-3-phosphocholine (1-acyl-2-acetyl-GPC) by different human inflammatory cells?
A: Yes, human inflammatory cells exhibit cell-type specific differences in the synthesis of PAF and 1-acyl-2-acetyl-GPC. While most inflammatory cells, except platelets, can synthesize both molecules, some cells like mast cells, basophils, and endothelial cells predominantly produce 1-acyl-2-acetyl-GPC. In contrast, neutrophils, eosinophils, and lung macrophages primarily synthesize PAF. This suggests distinct roles for these two phospholipids in various inflammatory processes. []
Q7: What are the advantages of using phospholipid-detergent conjugates for gene delivery?
A: Phospholipid-detergent conjugates offer advantages as fusogenic carriers for gene delivery. Studies using 1,2-dioleoyl-sn-glycero-3-phosphocholine (DOPC) conjugated with detergent C12E4 demonstrated efficient DNA delivery to cells with minimal toxicity. The fusogenic properties, crucial for intracellular delivery, were directly correlated with the length of the detergent's polyether moiety. In vivo experiments showed DOPC-C12E4's high potency for lung gene delivery, making it a promising alternative to existing methods. []
Q8: How can mass spectrometry be utilized to study phospholipid oxidation?
A: Field-induced droplet ionization mass spectrometry (FIDI-MS) enables the direct measurement of chemical degradation in phospholipid monolayers at the air-water interface. By exposing DPPC and POPG monolayers to reactive species like OH radicals and HCl vapor, FIDI-MS allows researchers to quantify the extent of lipid oxidation and identify specific reaction products, providing insights into the chemoprotective role of cholesterol in these systems. []
Q9: What is the role of ammonium salts in electrospray ionization mass spectrometry of phosphatidylcholines?
A: While ammonium salts like ammonium acetate are commonly added to the mobile phase during electrospray ionization mass spectrometry of phosphatidylcholines (PCs), their role is not to directly protonate the PC molecule. Instead, experiments using deuterated ammonium acetate demonstrated that water acts as the proton source, forming [M + D]+ ions. Adding ammonium salts or organic acids can even suppress PC ionization in some cases. []
体外研究产品的免责声明和信息
请注意,BenchChem 上展示的所有文章和产品信息仅供信息参考。 BenchChem 上可购买的产品专为体外研究设计,这些研究在生物体外进行。体外研究,源自拉丁语 "in glass",涉及在受控实验室环境中使用细胞或组织进行的实验。重要的是要注意,这些产品没有被归类为药物或药品,他们没有得到 FDA 的批准,用于预防、治疗或治愈任何医疗状况、疾病或疾病。我们必须强调,将这些产品以任何形式引入人类或动物的身体都是法律严格禁止的。遵守这些指南对确保研究和实验的法律和道德标准的符合性至关重要。