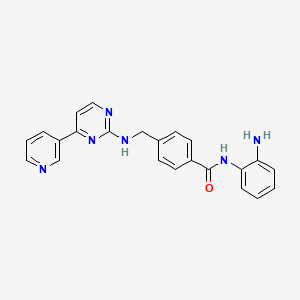
Mocetinostat
描述
Mocetinostat is a benzamide histone deacetylase inhibitor that is currently undergoing clinical trials for the treatment of various cancers, including follicular lymphoma, Hodgkin’s lymphoma, and acute myelogenous leukemia . It works by inhibiting histone deacetylase enzymes, which play a crucial role in the regulation of gene expression .
作用机制
Target of Action
Mocetinostat, also known as MGCD0103, is a novel isotypic-selective inhibitor of the enzyme histone deacetylase (HDAC) . It primarily targets HDAC1, but also inhibits HDAC2, HDAC3, and HDAC11 . These enzymes play a crucial role in the regulation of gene expression by removing acetyl groups from histones, which are proteins that help package DNA into a compact, organized structure within the cell nucleus .
Mode of Action
HDAC inhibitors like this compound act by turning on tumour suppressor genes that have been inappropriately turned off . Tumour suppressor genes are a natural defense against cancer. By inhibiting HDACs, this compound may restore normal cell function and reduce or inhibit tumour growth .
Biochemical Pathways
The inhibition of HDACs by this compound leads to the hyperacetylation of histone H3 . This hyperacetylation correlates with the inhibition of proliferation, induction of cell differentiation, and apoptosis . These changes can affect a variety of molecular processes, including pancreatic cell destiny, insulin release, insulin production, insulin signalling, and glucose metabolism .
Pharmacokinetics
Clinical trials have shown that this compound is well-tolerated, with favorable pharmacokinetics and pharmacodynamics .
Result of Action
The result of this compound’s action is the induction of tumor cell death . This may occur through various mechanisms, including the induction of apoptosis, differentiation, cell cycle arrest, inhibition of DNA repair, upregulation of tumor suppressors, downregulation of growth factors, oxidative stress, and autophagy .
Action Environment
Environmental factors, including nutrition, toxicants, and ethanol, can influence the action, efficacy, and stability of this compound . These factors can induce epigenetic modifications, such as DNA methylation, histone deacetylation, and miRNA regulation . Studying these mechanisms can facilitate the development of early diagnostic strategies and potential therapeutic avenues .
生化分析
Biochemical Properties
Mocetinostat plays a crucial role in biochemical reactions by inhibiting specific HDAC enzymes. It selectively inhibits HDAC1, HDAC2, HDAC3, and HDAC11, with IC50 values of 0.15, 0.29, 1.66, and 0.59 μM, respectively . This inhibition leads to the accumulation of acetylated histones, which can alter gene expression. This compound interacts with various proteins and enzymes, including HDACs, to modulate chromatin structure and function.
Cellular Effects
This compound exerts significant effects on various cell types and cellular processes. It influences cell function by altering cell signaling pathways, gene expression, and cellular metabolism. For instance, in cancer cells, this compound has been shown to induce cell cycle arrest and apoptosis by modulating the expression of genes involved in these processes . Additionally, it can affect cellular metabolism by altering the acetylation status of metabolic enzymes.
Molecular Mechanism
The molecular mechanism of this compound involves its binding to the active site of HDAC enzymes, thereby inhibiting their deacetylase activity. This inhibition leads to an increase in acetylated histones, which can affect the transcription of genes involved in cell cycle regulation, apoptosis, and differentiation . This compound also influences non-histone proteins, further contributing to its broad range of cellular effects.
Temporal Effects in Laboratory Settings
In laboratory settings, the effects of this compound have been observed to change over time. The compound is relatively stable, but its activity can decrease due to degradation. Long-term studies have shown that continuous exposure to this compound can lead to sustained changes in gene expression and cellular function . These temporal effects are crucial for understanding the long-term implications of this compound treatment.
Dosage Effects in Animal Models
The effects of this compound vary with different dosages in animal models. At lower doses, this compound can effectively inhibit HDAC activity without causing significant toxicity. At higher doses, toxic effects such as weight loss, hematological changes, and organ toxicity have been observed . Understanding the dosage effects is essential for optimizing therapeutic regimens and minimizing adverse effects.
Metabolic Pathways
This compound is involved in several metabolic pathways, primarily through its interaction with HDAC enzymes. By inhibiting these enzymes, this compound can alter the acetylation status of various metabolic enzymes, affecting metabolic flux and metabolite levels . This can lead to changes in cellular energy production and utilization, impacting overall cellular metabolism.
Transport and Distribution
Within cells and tissues, this compound is transported and distributed through various mechanisms. It can interact with transporters and binding proteins that facilitate its uptake and localization. This compound tends to accumulate in the nucleus, where it exerts its primary effects on chromatin and gene expression . Its distribution is crucial for its efficacy and specificity in targeting HDACs.
Subcellular Localization
This compound predominantly localizes to the nucleus, where it targets HDAC enzymes associated with chromatin. This subcellular localization is facilitated by specific targeting signals and post-translational modifications that direct this compound to the nucleus . The nuclear localization is essential for its function in modulating histone acetylation and gene expression.
准备方法
Synthetic Routes and Reaction Conditions: Mocetinostat is synthesized through a multi-step process involving the formation of a benzamide core structure. The synthesis typically starts with the reaction of 2-aminobenzamide with 4-(pyridin-3-yl)pyrimidin-2-ylamine under specific conditions to form the desired product .
Industrial Production Methods: The industrial production of this compound involves optimizing the reaction conditions to ensure high yield and purity. This includes controlling the temperature, pH, and reaction time to achieve the desired product efficiently .
化学反应分析
Types of Reactions: Mocetinostat undergoes various chemical reactions, including:
Oxidation: It can be oxidized under specific conditions to form different oxidation products.
Reduction: Reduction reactions can modify the functional groups present in this compound.
Substitution: Substitution reactions can occur at various positions on the benzamide core.
Common Reagents and Conditions:
Oxidation: Common oxidizing agents include hydrogen peroxide and potassium permanganate.
Reduction: Reducing agents such as sodium borohydride and lithium aluminum hydride are used.
Substitution: Substitution reactions often involve halogenating agents and nucleophiles.
Major Products Formed: The major products formed from these reactions depend on the specific conditions and reagents used. For example, oxidation can lead to the formation of hydroxylated derivatives, while reduction can produce amine derivatives .
科学研究应用
Mocetinostat has a wide range of scientific research applications, including:
相似化合物的比较
Vorinostat: Another histone deacetylase inhibitor used in the treatment of cutaneous T-cell lymphoma.
Romidepsin: A histone deacetylase inhibitor used for the treatment of peripheral T-cell lymphoma.
Panobinostat: A histone deacetylase inhibitor used in combination with other drugs for the treatment of multiple myeloma.
Uniqueness of Mocetinostat: this compound is unique in its selective inhibition of specific histone deacetylase enzymes, which allows for targeted therapeutic effects with potentially fewer side effects compared to other histone deacetylase inhibitors .
属性
IUPAC Name |
N-(2-aminophenyl)-4-[[(4-pyridin-3-ylpyrimidin-2-yl)amino]methyl]benzamide | |
---|---|---|
Source | PubChem | |
URL | https://pubchem.ncbi.nlm.nih.gov | |
Description | Data deposited in or computed by PubChem | |
InChI |
InChI=1S/C23H20N6O/c24-19-5-1-2-6-21(19)28-22(30)17-9-7-16(8-10-17)14-27-23-26-13-11-20(29-23)18-4-3-12-25-15-18/h1-13,15H,14,24H2,(H,28,30)(H,26,27,29) | |
Source | PubChem | |
URL | https://pubchem.ncbi.nlm.nih.gov | |
Description | Data deposited in or computed by PubChem | |
InChI Key |
HRNLUBSXIHFDHP-UHFFFAOYSA-N | |
Source | PubChem | |
URL | https://pubchem.ncbi.nlm.nih.gov | |
Description | Data deposited in or computed by PubChem | |
Canonical SMILES |
C1=CC=C(C(=C1)N)NC(=O)C2=CC=C(C=C2)CNC3=NC=CC(=N3)C4=CN=CC=C4 | |
Source | PubChem | |
URL | https://pubchem.ncbi.nlm.nih.gov | |
Description | Data deposited in or computed by PubChem | |
Molecular Formula |
C23H20N6O | |
Source | PubChem | |
URL | https://pubchem.ncbi.nlm.nih.gov | |
Description | Data deposited in or computed by PubChem | |
DSSTOX Substance ID |
DTXSID80222945 | |
Record name | Mocetinostat | |
Source | EPA DSSTox | |
URL | https://comptox.epa.gov/dashboard/DTXSID80222945 | |
Description | DSSTox provides a high quality public chemistry resource for supporting improved predictive toxicology. | |
Molecular Weight |
396.4 g/mol | |
Source | PubChem | |
URL | https://pubchem.ncbi.nlm.nih.gov | |
Description | Data deposited in or computed by PubChem | |
Physical Description |
Solid; [Sigma-Aldrich MSDS] | |
Record name | Peroxidase | |
Source | Haz-Map, Information on Hazardous Chemicals and Occupational Diseases | |
URL | https://haz-map.com/Agents/16483 | |
Description | Haz-Map® is an occupational health database designed for health and safety professionals and for consumers seeking information about the adverse effects of workplace exposures to chemical and biological agents. | |
Explanation | Copyright (c) 2022 Haz-Map(R). All rights reserved. Unless otherwise indicated, all materials from Haz-Map are copyrighted by Haz-Map(R). No part of these materials, either text or image may be used for any purpose other than for personal use. Therefore, reproduction, modification, storage in a retrieval system or retransmission, in any form or by any means, electronic, mechanical or otherwise, for reasons other than personal use, is strictly prohibited without prior written permission. | |
Mechanism of Action |
Mocetinostat is a novel isotypic-selective inhibitor of the enzyme histone deacetylase (HDAC). HDAC inhibitors act by turning on tumour suppressor genes that have been inappropriately turned off. Tumour suppressor genes are a natural defense against cancer. It is therefore hypothesized that specifically inhibiting those HDACs involved in cancer with Mocetinostat may restore normal cell function and reduce or inhibit tumour growth. | |
Record name | Mocetinostat | |
Source | DrugBank | |
URL | https://www.drugbank.ca/drugs/DB11830 | |
Description | The DrugBank database is a unique bioinformatics and cheminformatics resource that combines detailed drug (i.e. chemical, pharmacological and pharmaceutical) data with comprehensive drug target (i.e. sequence, structure, and pathway) information. | |
Explanation | Creative Common's Attribution-NonCommercial 4.0 International License (http://creativecommons.org/licenses/by-nc/4.0/legalcode) | |
CAS No. |
726169-73-9, 9003-99-0 | |
Record name | Mocetinostat | |
Source | CAS Common Chemistry | |
URL | https://commonchemistry.cas.org/detail?cas_rn=726169-73-9 | |
Description | CAS Common Chemistry is an open community resource for accessing chemical information. Nearly 500,000 chemical substances from CAS REGISTRY cover areas of community interest, including common and frequently regulated chemicals, and those relevant to high school and undergraduate chemistry classes. This chemical information, curated by our expert scientists, is provided in alignment with our mission as a division of the American Chemical Society. | |
Explanation | The data from CAS Common Chemistry is provided under a CC-BY-NC 4.0 license, unless otherwise stated. | |
Record name | Mocetinostat [USAN:INN] | |
Source | ChemIDplus | |
URL | https://pubchem.ncbi.nlm.nih.gov/substance/?source=chemidplus&sourceid=0726169739 | |
Description | ChemIDplus is a free, web search system that provides access to the structure and nomenclature authority files used for the identification of chemical substances cited in National Library of Medicine (NLM) databases, including the TOXNET system. | |
Record name | Mocetinostat | |
Source | DrugBank | |
URL | https://www.drugbank.ca/drugs/DB11830 | |
Description | The DrugBank database is a unique bioinformatics and cheminformatics resource that combines detailed drug (i.e. chemical, pharmacological and pharmaceutical) data with comprehensive drug target (i.e. sequence, structure, and pathway) information. | |
Explanation | Creative Common's Attribution-NonCommercial 4.0 International License (http://creativecommons.org/licenses/by-nc/4.0/legalcode) | |
Record name | Peroxidase | |
Source | EPA Chemicals under the TSCA | |
URL | https://www.epa.gov/chemicals-under-tsca | |
Description | EPA Chemicals under the Toxic Substances Control Act (TSCA) collection contains information on chemicals and their regulations under TSCA, including non-confidential content from the TSCA Chemical Substance Inventory and Chemical Data Reporting. | |
Record name | Mocetinostat | |
Source | EPA DSSTox | |
URL | https://comptox.epa.gov/dashboard/DTXSID80222945 | |
Description | DSSTox provides a high quality public chemistry resource for supporting improved predictive toxicology. | |
Record name | Peroxidase | |
Source | European Chemicals Agency (ECHA) | |
URL | https://echa.europa.eu/substance-information/-/substanceinfo/100.029.686 | |
Description | The European Chemicals Agency (ECHA) is an agency of the European Union which is the driving force among regulatory authorities in implementing the EU's groundbreaking chemicals legislation for the benefit of human health and the environment as well as for innovation and competitiveness. | |
Explanation | Use of the information, documents and data from the ECHA website is subject to the terms and conditions of this Legal Notice, and subject to other binding limitations provided for under applicable law, the information, documents and data made available on the ECHA website may be reproduced, distributed and/or used, totally or in part, for non-commercial purposes provided that ECHA is acknowledged as the source: "Source: European Chemicals Agency, http://echa.europa.eu/". Such acknowledgement must be included in each copy of the material. ECHA permits and encourages organisations and individuals to create links to the ECHA website under the following cumulative conditions: Links can only be made to webpages that provide a link to the Legal Notice page. | |
Record name | MOCETINOSTAT | |
Source | FDA Global Substance Registration System (GSRS) | |
URL | https://gsrs.ncats.nih.gov/ginas/app/beta/substances/A6GWB8T96J | |
Description | The FDA Global Substance Registration System (GSRS) enables the efficient and accurate exchange of information on what substances are in regulated products. Instead of relying on names, which vary across regulatory domains, countries, and regions, the GSRS knowledge base makes it possible for substances to be defined by standardized, scientific descriptions. | |
Explanation | Unless otherwise noted, the contents of the FDA website (www.fda.gov), both text and graphics, are not copyrighted. They are in the public domain and may be republished, reprinted and otherwise used freely by anyone without the need to obtain permission from FDA. Credit to the U.S. Food and Drug Administration as the source is appreciated but not required. | |
Retrosynthesis Analysis
AI-Powered Synthesis Planning: Our tool employs the Template_relevance Pistachio, Template_relevance Bkms_metabolic, Template_relevance Pistachio_ringbreaker, Template_relevance Reaxys, Template_relevance Reaxys_biocatalysis model, leveraging a vast database of chemical reactions to predict feasible synthetic routes.
One-Step Synthesis Focus: Specifically designed for one-step synthesis, it provides concise and direct routes for your target compounds, streamlining the synthesis process.
Accurate Predictions: Utilizing the extensive PISTACHIO, BKMS_METABOLIC, PISTACHIO_RINGBREAKER, REAXYS, REAXYS_BIOCATALYSIS database, our tool offers high-accuracy predictions, reflecting the latest in chemical research and data.
Strategy Settings
Precursor scoring | Relevance Heuristic |
---|---|
Min. plausibility | 0.01 |
Model | Template_relevance |
Template Set | Pistachio/Bkms_metabolic/Pistachio_ringbreaker/Reaxys/Reaxys_biocatalysis |
Top-N result to add to graph | 6 |
Feasible Synthetic Routes
Q1: What is peroxidase, and what is its primary function?
A: Peroxidases are a large family of enzymes that catalyze the oxidation of a variety of organic and inorganic substrates using hydrogen peroxide (H2O2) as an electron acceptor [, , , , ]. These enzymes play crucial roles in various biological processes, including detoxification of reactive oxygen species, biosynthesis of lignin and other cell wall components, and defense against pathogens [, , , , ].
Q2: How do peroxidases interact with their substrates?
A: Peroxidases typically contain a heme prosthetic group, often iron protoporphyrin IX, at their active site [, ]. The catalytic cycle involves a two-electron oxidation of the enzyme by hydrogen peroxide, forming a highly reactive intermediate called Compound I. Compound I then oxidizes the substrate by one electron, generating a substrate radical and Compound II. Finally, Compound II oxidizes another substrate molecule, returning the enzyme to its resting state [, , ].
Q3: What are the downstream effects of peroxidase activity?
A: The downstream effects of peroxidase activity are highly dependent on the specific enzyme and substrate involved. For example, in plants, peroxidases contribute to lignin biosynthesis, strengthening cell walls and providing resistance against pathogens [, ]. In mammals, glutathione peroxidase utilizes glutathione to reduce hydrogen peroxide to water, protecting cells from oxidative damage [].
Q4: Can you provide information about the structural characterization of peroxidases?
A: The specific molecular formula and weight of peroxidases vary depending on the source and isoform. For example, horseradish peroxidase (HRP), a commonly studied peroxidase, has a molecular weight of approximately 44 kDa []. Spectroscopic techniques like UV-Vis, circular dichroism, and resonance Raman spectroscopy are frequently employed to characterize the heme environment and structural changes in peroxidases [, , ].
Q5: How do different factors influence the stability and activity of peroxidases?
A: Peroxidase activity and stability are influenced by various factors like pH, temperature, and the presence of inhibitors or activators [, , ]. For instance, tobacco peroxidase exhibits enhanced stability at acidic pH in the presence of calcium cations [], while bovine lactoperoxidase activity is stabilized by the cationic surfactant benzalkonium chloride [].
Q6: What are some known applications of peroxidases?
A: Peroxidases find applications in various fields due to their catalytic properties. They are used in diagnostic assays, like ELISA, for signal amplification []. In bioremediation, fungal peroxidases, such as lignin peroxidase, contribute to the degradation of lignin and other pollutants [, ]. Moreover, peroxidases are being explored for potential use in biocatalysis, biosensing, and biofuel production [, , ].
Q7: How does computational chemistry contribute to peroxidase research?
A: Computational chemistry plays a vital role in understanding peroxidase structure, function, and mechanism. Molecular modeling and simulations can provide insights into substrate binding, catalytic mechanisms, and the impact of mutations on enzyme activity [, ]. Additionally, quantitative structure-activity relationship (QSAR) models can be developed to predict the activity and properties of novel peroxidase substrates or inhibitors [].
Q8: How does the structure of a peroxidase influence its activity and selectivity?
A: The structure of a peroxidase, particularly the architecture of its active site, significantly influences its activity and substrate specificity []. Mutations or modifications in the distal histidine, a crucial residue in the active site, can significantly alter peroxidase activity, as demonstrated with horseradish peroxidase mutants [, ]. Furthermore, the size and shape of the substrate-binding pocket dictate the range of substrates a peroxidase can accommodate [].
Q9: What are some strategies for improving the stability and formulation of peroxidases?
A: Various strategies are employed to enhance peroxidase stability and facilitate their use in different applications. Immobilization on solid supports, such as silica-titanium oxide or poly(l-lysine) films, can significantly enhance enzyme stability and enable their use at elevated temperatures [, ]. Additionally, the addition of stabilizers, like surfactants or polyols, can protect peroxidases from denaturation and improve their shelf life [].
Q10: Are there any safety regulations concerning the use of peroxidases?
A: While peroxidases are generally considered safe for many applications, appropriate safety guidelines should be followed when handling and using these enzymes, especially in industrial settings. Depending on the application, regulatory agencies like the FDA or EPA may have specific guidelines regarding the use and disposal of peroxidase-containing products [].
Q11: Can you provide information about peroxidase levels in relation to disease?
A: Altered peroxidase activity has been linked to various diseases, although the specific role of peroxidases in disease pathogenesis remains an area of active research. For example, decreased glutathione peroxidase activity has been observed in patients with chronic pancreatitis and oral cavity malignancies [, ]. Conversely, increased peroxidase activity has been reported in certain cancers and inflammatory conditions [, , ].
Q12: What are some challenges and future directions in peroxidase research?
A: Despite significant advances in peroxidase research, several challenges and opportunities remain. Developing highly active and stable peroxidases for industrial applications, understanding the complex interplay of peroxidases in various biological processes, and exploring their therapeutic potential for treating diseases are just a few areas where further research is needed [, , , , , ].
体外研究产品的免责声明和信息
请注意,BenchChem 上展示的所有文章和产品信息仅供信息参考。 BenchChem 上可购买的产品专为体外研究设计,这些研究在生物体外进行。体外研究,源自拉丁语 "in glass",涉及在受控实验室环境中使用细胞或组织进行的实验。重要的是要注意,这些产品没有被归类为药物或药品,他们没有得到 FDA 的批准,用于预防、治疗或治愈任何医疗状况、疾病或疾病。我们必须强调,将这些产品以任何形式引入人类或动物的身体都是法律严格禁止的。遵守这些指南对确保研究和实验的法律和道德标准的符合性至关重要。