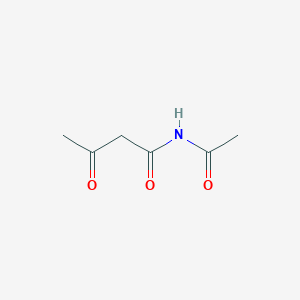
N-acetyl-3-oxobutanamide
- 点击 快速询问 获取最新报价。
- 提供有竞争力价格的高质量产品,您可以更专注于研究。
描述
作用机制
Target of Action
N-acetyl-3-oxobutanamide primarily targets the flavoHB enzyme, which is a well-validated target for the identification of antibacterial inhibitors . This enzyme plays a crucial role in the biochemical reactions of bacteria, making it a promising target for antimicrobial agents .
Mode of Action
It is known that this compound interacts with its target, the flavohb enzyme, to inhibit its function . This interaction results in the disruption of the enzyme’s normal activity, leading to the inhibition of bacterial growth .
Biochemical Pathways
This compound is involved in the hexosamine biosynthesis pathway (HBP), which utilizes substrates including fructose-6-phosphate, glutamine, acetyl-coenzyme A, and uridine triphosphate to synthesize uridine diphosphate-N-acetylglucosamine . This pathway plays a crucial role in the normal growth and development of organisms .
Result of Action
The result of this compound’s action is the inhibition of bacterial growth. By interacting with the flavoHB enzyme, this compound disrupts the normal biochemical reactions of bacteria, leading to the inhibition of their growth .
生化分析
Biochemical Properties
N-acetyl-3-oxobutanamide plays a crucial role in several biochemical reactions. It interacts with various enzymes, proteins, and other biomolecules. One of the key interactions is with N-terminal acetyltransferases (NATs), which acetylate the N-terminus of proteins, affecting their stability, localization, and interactions . This compound also interacts with enzymes involved in oxidative stress responses, such as glutathione peroxidase, which helps in reducing oxidative damage .
Cellular Effects
This compound influences various cellular processes, including cell signaling pathways, gene expression, and cellular metabolism. It has been shown to modulate the activity of key signaling molecules like c-Jun N-terminal kinase and p38 MAP kinase, which are involved in stress responses . Additionally, this compound affects gene expression by regulating transcription factors such as nuclear factor kappa B, leading to changes in the expression of genes involved in inflammation and cell survival .
Molecular Mechanism
The molecular mechanism of action of this compound involves its interaction with various biomolecules. It acts as a precursor for the synthesis of glutathione, an important antioxidant that protects cells from oxidative damage . This compound also inhibits the activity of certain enzymes, such as acetyl-CoA carboxylase, which plays a role in fatty acid metabolism . These interactions result in changes in cellular processes and gene expression, contributing to its overall effects.
Temporal Effects in Laboratory Settings
In laboratory settings, the effects of this compound can change over time. Studies have shown that its stability and degradation can influence its long-term effects on cellular function. For example, prolonged exposure to this compound can lead to sustained activation of antioxidant pathways and protection against oxidative stress . Its stability may vary depending on the experimental conditions, such as temperature and pH .
Dosage Effects in Animal Models
The effects of this compound vary with different dosages in animal models. At lower doses, it has been shown to provide protective effects against oxidative stress and inflammation . At higher doses, it may exhibit toxic effects, such as liver and kidney damage . The optimal dosage for achieving therapeutic effects without adverse effects needs to be carefully determined in animal studies.
Metabolic Pathways
This compound is involved in several metabolic pathways. It is metabolized by enzymes such as acetyl-CoA synthetase and acetyl-CoA carboxylase, which play roles in fatty acid metabolism and energy production . Additionally, it can be converted into other metabolites, such as N-acetyl-S-(2-carboxypropyl)cysteine, which is involved in detoxification processes .
Transport and Distribution
Within cells and tissues, this compound is transported and distributed through various mechanisms. It can diffuse across cell membranes and interact with transporters and binding proteins that facilitate its movement . The distribution of this compound within tissues can affect its localization and accumulation, influencing its overall activity and function .
Subcellular Localization
The subcellular localization of this compound is crucial for its activity and function. It can be targeted to specific compartments or organelles through post-translational modifications and targeting signals . For example, this compound may localize to the mitochondria, where it can participate in antioxidant defense and energy metabolism . Its localization can also affect its interactions with other biomolecules and its overall cellular effects.
准备方法
Synthetic Routes and Reaction Conditions: N-acetyl-3-oxobutanamide can be synthesized through several methods. One common approach involves the reaction of acetoacetic acid with acetic anhydride in the presence of a catalyst. The reaction typically proceeds under mild conditions, yielding this compound as the primary product.
Industrial Production Methods: In an industrial setting, the production of this compound often involves the use of large-scale reactors and optimized reaction conditions to ensure high yield and purity. The process may include steps such as purification through recrystallization or distillation to remove any impurities.
化学反应分析
Types of Reactions: N-acetyl-3-oxobutanamide undergoes various chemical reactions, including:
Oxidation: The compound can be oxidized to form corresponding carboxylic acids.
Reduction: Reduction reactions can convert the ketone group to an alcohol.
Substitution: The amide group can participate in nucleophilic substitution reactions.
Common Reagents and Conditions:
Oxidation: Common oxidizing agents include potassium permanganate and chromium trioxide.
Reduction: Reducing agents such as sodium borohydride or lithium aluminum hydride are typically used.
Substitution: Nucleophiles like amines or alcohols can be used under basic or acidic conditions.
Major Products Formed:
Oxidation: Acetoacetic acid derivatives.
Reduction: Hydroxy derivatives.
Substitution: Various substituted amides or esters.
科学研究应用
N-acetyl-3-oxobutanamide has a wide range of applications in scientific research:
Chemistry: It is used as a building block in organic synthesis and as a reagent in various chemical reactions.
Biology: The compound is studied for its potential biological activities, including antimicrobial and anti-inflammatory properties.
Medicine: Research is ongoing to explore its potential therapeutic applications, particularly in drug development.
Industry: this compound is used in the production of pharmaceuticals, agrochemicals, and other fine chemicals.
相似化合物的比较
Acetoacetamide: Similar in structure but lacks the acetyl group.
Acetylacetone: Contains a similar diketone structure but lacks the amide group.
N-acetyl-2-oxobutanamide: A structural isomer with different positioning of functional groups.
Uniqueness: N-acetyl-3-oxobutanamide is unique due to the presence of both an amide and a ketone functional group, which imparts distinct chemical reactivity and potential for diverse applications. Its ability to participate in a variety of chemical reactions makes it a valuable compound in research and industry.
生物活性
N-acetyl-3-oxobutanamide is a compound of interest in medicinal chemistry, particularly due to its potential biological activities. This article will explore its biological activity, mechanisms of action, and relevant research findings, including data tables and case studies.
Antimicrobial Properties
Research indicates that this compound exhibits significant antimicrobial activity. In vitro studies have demonstrated its effectiveness against various bacterial strains, including Escherichia coli and Bacillus subtilis. The compound's mechanism involves the inhibition of bacterial growth through interference with essential metabolic pathways.
Table 1: Antimicrobial Activity of this compound
Bacterial Strain | Minimum Inhibitory Concentration (MIC) | Reference |
---|---|---|
Escherichia coli | 50 µg/mL | |
Bacillus subtilis | 40 µg/mL |
Anticancer Properties
This compound has also been investigated for its anticancer properties. Studies have shown that it can induce apoptosis in cancer cell lines, potentially through the activation of caspases and modulation of apoptotic pathways. For instance, a study conducted on human breast cancer cells demonstrated that treatment with the compound led to a significant reduction in cell viability.
Case Study: Induction of Apoptosis in Breast Cancer Cells
A study published in 2023 evaluated the effects of this compound on MCF-7 breast cancer cells. The results indicated:
- Cell Viability : A reduction to 30% of control after 48 hours of treatment.
- Caspase Activation : Increased levels of active caspase-3 and caspase-9 were observed.
These findings suggest that this compound may act as a potential chemotherapeutic agent by promoting programmed cell death in cancer cells.
Enzyme Inhibition
The compound has shown promise as an enzyme inhibitor, particularly against enzymes involved in carbohydrate metabolism. For example, it has been reported to inhibit α-amylase activity, which is crucial for starch digestion.
Table 2: Enzyme Inhibition Activity
This inhibition could have implications for managing conditions such as diabetes by regulating glucose absorption.
The biological effects of this compound are attributed to its interaction with specific molecular targets. The compound can inhibit certain enzymes or receptors, leading to its antimicrobial and anticancer effects.
- Antimicrobial Action : Disruption of bacterial cell wall synthesis and metabolic pathways.
- Anticancer Mechanism : Induction of apoptosis via caspase activation and modulation of signaling pathways.
- Enzyme Inhibition : Competitive inhibition at the active sites of target enzymes like α-amylase.
属性
IUPAC Name |
N-acetyl-3-oxobutanamide |
Source
|
---|---|---|
Details | Computed by LexiChem 2.6.6 (PubChem release 2019.06.18) | |
Source | PubChem | |
URL | https://pubchem.ncbi.nlm.nih.gov | |
Description | Data deposited in or computed by PubChem | |
InChI |
InChI=1S/C6H9NO3/c1-4(8)3-6(10)7-5(2)9/h3H2,1-2H3,(H,7,9,10) |
Source
|
Details | Computed by InChI 1.0.5 (PubChem release 2019.06.18) | |
Source | PubChem | |
URL | https://pubchem.ncbi.nlm.nih.gov | |
Description | Data deposited in or computed by PubChem | |
InChI Key |
IZOZBUJRWPPEBT-UHFFFAOYSA-N |
Source
|
Details | Computed by InChI 1.0.5 (PubChem release 2019.06.18) | |
Source | PubChem | |
URL | https://pubchem.ncbi.nlm.nih.gov | |
Description | Data deposited in or computed by PubChem | |
Canonical SMILES |
CC(=O)CC(=O)NC(=O)C |
Source
|
Details | Computed by OEChem 2.1.5 (PubChem release 2019.06.18) | |
Source | PubChem | |
URL | https://pubchem.ncbi.nlm.nih.gov | |
Description | Data deposited in or computed by PubChem | |
Molecular Formula |
C6H9NO3 |
Source
|
Details | Computed by PubChem 2.1 (PubChem release 2019.06.18) | |
Source | PubChem | |
URL | https://pubchem.ncbi.nlm.nih.gov | |
Description | Data deposited in or computed by PubChem | |
Molecular Weight |
143.14 g/mol |
Source
|
Details | Computed by PubChem 2.1 (PubChem release 2021.05.07) | |
Source | PubChem | |
URL | https://pubchem.ncbi.nlm.nih.gov | |
Description | Data deposited in or computed by PubChem | |
体外研究产品的免责声明和信息
请注意,BenchChem 上展示的所有文章和产品信息仅供信息参考。 BenchChem 上可购买的产品专为体外研究设计,这些研究在生物体外进行。体外研究,源自拉丁语 "in glass",涉及在受控实验室环境中使用细胞或组织进行的实验。重要的是要注意,这些产品没有被归类为药物或药品,他们没有得到 FDA 的批准,用于预防、治疗或治愈任何医疗状况、疾病或疾病。我们必须强调,将这些产品以任何形式引入人类或动物的身体都是法律严格禁止的。遵守这些指南对确保研究和实验的法律和道德标准的符合性至关重要。