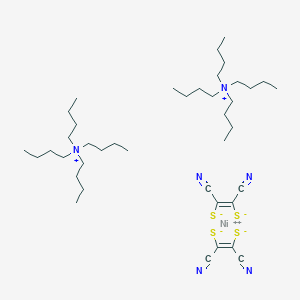
双(马来腈二硫醇)镍(2+)双(四丁基氮); Bis(tetra-n-butylammonium) bis(maleonitriledithiolato) nickel complex
- 点击 快速询问 获取最新报价。
- 提供有竞争力价格的高质量产品,您可以更专注于研究。
描述
(Z)-1,2-dicyanoethene-1,2-dithiolate;nickel(2+);tetrabutylazanium is a coordination compound with the molecular formula C40H72N6NiS4. It is known for its unique properties and applications in various fields of scientific research. The compound is characterized by its orange to red powder or crystalline appearance and has a molecular weight of 824 g/mol .
科学研究应用
(Z)-1,2-dicyanoethene-1,2-dithiolate;nickel(2+);tetrabutylazanium has a wide range of applications in scientific research:
Catalysis: It is used as a precursor for nickel-based catalysts in various organic transformations.
Magnetism: The compound exhibits unique magnetic properties, making it useful in the study of magnetic materials.
Electron Paramagnetic Resonance (EPR) Spectroscopy: It serves as a spin trapping agent in EPR spectroscopy for studying free radicals.
Material Science: The compound’s unique crystal structure and thermal stability make it valuable in material science research.
作用机制
Target of Action
The compound is a quaternary ammonium salt, and such compounds are generally known to interact with a variety of biological targets, including enzymes and cell membranes .
Mode of Action
Quaternary ammonium compounds are known to interact with their targets through a variety of mechanisms, including disruption of cell membrane integrity and inhibition of enzyme activity . The specific interactions of this compound with its targets would depend on the chemical structure of the compound and the nature of the target.
Biochemical Pathways
Quaternary ammonium compounds can influence a variety of biochemical processes, including those involved in energy production and cellular homeostasis .
Pharmacokinetics
The pharmacokinetics of quaternary ammonium compounds can vary widely depending on their chemical structure and the route of administration .
Result of Action
The action of quaternary ammonium compounds can result in a variety of effects, including cell death, inhibition of cellular processes, and changes in cell morphology .
准备方法
Synthetic Routes and Reaction Conditions
The synthesis of (Z)-1,2-dicyanoethene-1,2-dithiolate;nickel(2+);tetrabutylazanium typically involves the reaction of nickel salts with maleonitriledithiolato ligands in the presence of tetra-n-butylammonium cations. The reaction is carried out under controlled conditions to ensure the formation of the desired complex. The process involves the following steps:
- Dissolution of nickel salts in a suitable solvent such as methanol.
- Addition of maleonitriledithiolato ligands to the solution.
- Introduction of tetra-n-butylammonium cations to the reaction mixture.
- Stirring the mixture at a specific temperature and time to allow the formation of the complex.
- Isolation and purification of the complex through filtration and recrystallization .
Industrial Production Methods
Industrial production methods for this compound are similar to the laboratory synthesis but are scaled up to meet the demand. The process involves the use of larger reaction vessels, automated systems for precise control of reaction conditions, and advanced purification techniques to ensure high purity and yield of the final product .
化学反应分析
Types of Reactions
(Z)-1,2-dicyanoethene-1,2-dithiolate;nickel(2+);tetrabutylazanium undergoes various chemical reactions, including:
Oxidation: The compound can be oxidized to form higher oxidation state species.
Reduction: It can be reduced to lower oxidation state species.
Substitution: Ligands in the complex can be substituted with other ligands under specific conditions.
Common Reagents and Conditions
Oxidation: Common oxidizing agents such as hydrogen peroxide or potassium permanganate can be used.
Reduction: Reducing agents like sodium borohydride or lithium aluminum hydride are employed.
Substitution: Ligand substitution reactions often require the presence of other ligands and may be facilitated by heating or the use of catalysts.
Major Products Formed
The major products formed from these reactions depend on the specific reagents and conditions used. For example, oxidation may yield nickel(III) complexes, while reduction can produce nickel(I) species. Substitution reactions result in the formation of new coordination complexes with different ligands.
相似化合物的比较
Similar Compounds
- Bis(tetra-n-butylammonium) peroxydisulfate
- Bis(tetra-n-butylammonium) bis(maleonitriledithiolato) cobalt complex
- Bis(tetra-n-butylammonium) bis(maleonitriledithiolato) iron complex
Uniqueness
(Z)-1,2-dicyanoethene-1,2-dithiolate;nickel(2+);tetrabutylazanium stands out due to its specific coordination environment and the unique properties imparted by the maleonitriledithiolato ligands. Compared to similar compounds, it exhibits distinct magnetic and catalytic properties, making it particularly valuable in research areas such as catalysis and material science .
属性
IUPAC Name |
1,2-dicyanoethene-1,2-dithiolate;nickel(2+);tetrabutylazanium |
Source
|
---|---|---|
Details | Computed by LexiChem 2.6.6 (PubChem release 2019.06.18) | |
Source | PubChem | |
URL | https://pubchem.ncbi.nlm.nih.gov | |
Description | Data deposited in or computed by PubChem | |
InChI |
InChI=1S/2C16H36N.2C4H2N2S2.Ni/c2*1-5-9-13-17(14-10-6-2,15-11-7-3)16-12-8-4;2*5-1-3(7)4(8)2-6;/h2*5-16H2,1-4H3;2*7-8H;/q2*+1;;;+2/p-4 |
Source
|
Details | Computed by InChI 1.0.5 (PubChem release 2019.06.18) | |
Source | PubChem | |
URL | https://pubchem.ncbi.nlm.nih.gov | |
Description | Data deposited in or computed by PubChem | |
InChI Key |
KFDRXQXGGQXFNP-UHFFFAOYSA-J |
Source
|
Details | Computed by InChI 1.0.5 (PubChem release 2019.06.18) | |
Source | PubChem | |
URL | https://pubchem.ncbi.nlm.nih.gov | |
Description | Data deposited in or computed by PubChem | |
Canonical SMILES |
CCCC[N+](CCCC)(CCCC)CCCC.CCCC[N+](CCCC)(CCCC)CCCC.C(#N)C(=C(C#N)[S-])[S-].C(#N)C(=C(C#N)[S-])[S-].[Ni+2] |
Source
|
Details | Computed by OEChem 2.1.5 (PubChem release 2019.06.18) | |
Source | PubChem | |
URL | https://pubchem.ncbi.nlm.nih.gov | |
Description | Data deposited in or computed by PubChem | |
Molecular Formula |
C40H72N6NiS4 |
Source
|
Details | Computed by PubChem 2.1 (PubChem release 2019.06.18) | |
Source | PubChem | |
URL | https://pubchem.ncbi.nlm.nih.gov | |
Description | Data deposited in or computed by PubChem | |
DSSTOX Substance ID |
DTXSID50466016 |
Source
|
Record name | MolPort-035-785-124 | |
Source | EPA DSSTox | |
URL | https://comptox.epa.gov/dashboard/DTXSID50466016 | |
Description | DSSTox provides a high quality public chemistry resource for supporting improved predictive toxicology. | |
Molecular Weight |
824.0 g/mol |
Source
|
Details | Computed by PubChem 2.1 (PubChem release 2021.05.07) | |
Source | PubChem | |
URL | https://pubchem.ncbi.nlm.nih.gov | |
Description | Data deposited in or computed by PubChem | |
CAS No. |
18958-57-1 |
Source
|
Record name | MolPort-035-785-124 | |
Source | EPA DSSTox | |
URL | https://comptox.epa.gov/dashboard/DTXSID50466016 | |
Description | DSSTox provides a high quality public chemistry resource for supporting improved predictive toxicology. | |
体外研究产品的免责声明和信息
请注意,BenchChem 上展示的所有文章和产品信息仅供信息参考。 BenchChem 上可购买的产品专为体外研究设计,这些研究在生物体外进行。体外研究,源自拉丁语 "in glass",涉及在受控实验室环境中使用细胞或组织进行的实验。重要的是要注意,这些产品没有被归类为药物或药品,他们没有得到 FDA 的批准,用于预防、治疗或治愈任何医疗状况、疾病或疾病。我们必须强调,将这些产品以任何形式引入人类或动物的身体都是法律严格禁止的。遵守这些指南对确保研究和实验的法律和道德标准的符合性至关重要。