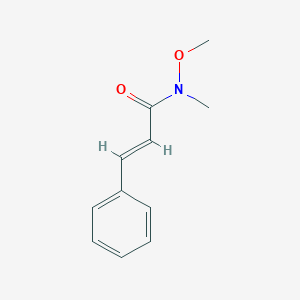
N-甲氧基-N-甲基肉桂酰胺
描述
N-Methoxy-N-methylcinnamamide is a chemical compound with the molecular formula C11H13NO2. It is a member of the class of compounds known as cinnamamides, which are derivatives of cinnamic acid. This compound is characterized by the presence of a methoxy group and a methyl group attached to the nitrogen atom of the amide functional group. N-Methoxy-N-methylcinnamamide is known for its applications in organic synthesis and medicinal chemistry.
科学研究应用
Synthetic Applications
1.1. Enzymatic Synthesis
Recent studies have highlighted the efficient synthesis of cinnamamide derivatives, including NMCA, using enzymatic methods. For instance, Lipozyme TL IM has been employed in continuous-flow microreactors to catalyze the conversion of methyl cinnamates and phenylethylamines into cinnamamide derivatives with high yields (up to 91.3%) under mild conditions . This method emphasizes the advantages of enzyme catalysis, such as short reaction times and environmental friendliness.
1.2. Chemical Reactions
NMCA has been utilized as a model compound for studying addition-elimination reactions in organic synthesis. Its structural properties allow for various modifications that can lead to the development of new compounds with desired functionalities . The compound's reactivity makes it a valuable intermediate in the synthesis of more complex molecules.
Pharmacological Properties
2.1. Antimicrobial Activity
Research has indicated that NMCA and its derivatives exhibit antimicrobial properties. For example, certain synthesized derivatives showed significant activity against various bacterial strains, suggesting potential applications in developing new antimicrobial agents . The structural features of NMCA contribute to its biological activity, making it a candidate for further pharmacological exploration.
2.2. Anticancer Potential
Preliminary studies have suggested that NMCA derivatives may possess anticancer properties. Investigations into the mechanism of action have revealed that these compounds can induce apoptosis in cancer cells, highlighting their potential as chemotherapeutic agents . Further research is needed to elucidate the specific pathways involved and to optimize their efficacy.
Case Studies
作用机制
Target of Action
N-Methoxy-N-methylcinnamamide is primarily used as a synthetic intermediate in organic synthesis . It is used in the Heck coupling of aryl halides . The primary targets of N-Methoxy-N-methylcinnamamide are therefore the aryl halides that it interacts with during the Heck coupling process .
Mode of Action
N-Methoxy-N-methylcinnamamide interacts with its targets, the aryl halides, through a Heck coupling process . This process involves the formation of a carbon-carbon bond between the aryl halide and N-Methoxy-N-methylcinnamamide . The result of this interaction is the formation of N-methoxy-N-methylcinnamamides .
Biochemical Pathways
The biochemical pathways affected by N-Methoxy-N-methylcinnamamide are those involved in the synthesis of aromatic α,β-unsaturated Weinreb amides . These compounds are widely used as flexible synthetic intermediates in organic synthesis . They afford rapid access to ketones on treatment with Grignard and organolithium reagents, and aldehydes with LiAlH4 .
Result of Action
The result of the action of N-Methoxy-N-methylcinnamamide is the formation of N-methoxy-N-methylcinnamamides . .
Action Environment
The action of N-Methoxy-N-methylcinnamamide is influenced by the environment in which the Heck coupling process takes place . The use of microwave irradiation can reduce reaction times from 3 hours (thermal) to 5–15 minutes with no substantive effect on reaction outcome . The reaction is also highly substrate tolerant with various moieties well tolerated .
准备方法
Synthetic Routes and Reaction Conditions
N-Methoxy-N-methylcinnamamide can be synthesized through various methods. One common approach involves the Heck coupling reaction of aryl halides with N-methoxy-N-methylacrylamide in the presence of a palladium catalyst. This reaction is typically carried out in room-temperature ionic liquids, such as [BMIM][PF6] or [BMIM][OH], which provide excellent yields and high purity . The use of microwave irradiation can significantly reduce reaction times from several hours to just a few minutes without affecting the reaction outcome .
Industrial Production Methods
In an industrial setting, the synthesis of N-Methoxy-N-methylcinnamamide may involve the use of large-scale reactors and optimized reaction conditions to ensure high yield and purity. The Heck coupling reaction remains a preferred method due to its efficiency and environmental friendliness compared to traditional acid chloride coupling methods .
化学反应分析
Types of Reactions
N-Methoxy-N-methylcinnamamide undergoes various chemical reactions, including:
Oxidation: This compound can be oxidized to form corresponding ketones or aldehydes.
Reduction: Reduction reactions can convert N-Methoxy-N-methylcinnamamide to its corresponding amine derivatives.
Substitution: The methoxy and methyl groups on the nitrogen atom can be substituted with other functional groups under appropriate conditions.
Common Reagents and Conditions
Oxidation: Common oxidizing agents include potassium permanganate (KMnO4) and chromium trioxide (CrO3).
Reduction: Reducing agents such as lithium aluminum hydride (LiAlH4) and sodium borohydride (NaBH4) are commonly used.
Substitution: Substitution reactions may involve reagents like alkyl halides or acyl chlorides.
Major Products Formed
The major products formed from these reactions depend on the specific reagents and conditions used. For example, oxidation may yield ketones or aldehydes, while reduction can produce amines.
相似化合物的比较
Similar Compounds
N-Methoxy-N-methylbenzamide: Similar in structure but lacks the cinnamoyl group.
N-Methoxy-N-methylacrylamide: Similar but with an acrylamide group instead of a cinnamamide group.
N-Methoxy-N-methylformamide: Similar but with a formamide group instead of a cinnamamide group.
Uniqueness
N-Methoxy-N-methylcinnamamide is unique due to the presence of the cinnamoyl group, which imparts specific reactivity and properties. This makes it particularly useful in the synthesis of cinnamaldehyde derivatives and other aromatic compounds .
生物活性
N-Methoxy-N-methylcinnamamide is a compound that has garnered attention in the field of medicinal chemistry due to its potential biological activities. This article reviews its synthesis, biological properties, and relevant case studies, supported by data tables and research findings.
Synthesis of N-Methoxy-N-methylcinnamamide
The synthesis of N-Methoxy-N-methylcinnamamide typically involves the reaction of methoxy and methyl groups with cinnamic acid derivatives. The compound can be synthesized using various methods, including the use of catalysts like ruthenium complexes, which facilitate the formation of amide bonds under mild conditions .
Biological Activity Overview
N-Methoxy-N-methylcinnamamide exhibits a range of biological activities that can be categorized as follows:
- Antiproliferative Activity : Several studies have investigated the antiproliferative effects of this compound against various cancer cell lines. The compound has shown significant activity, with IC50 values indicating its effectiveness in inhibiting cell growth.
- Antioxidant Properties : Research indicates that N-Methoxy-N-methylcinnamamide possesses antioxidant properties, contributing to its potential therapeutic applications .
- Antibacterial Effects : The compound has demonstrated selective antibacterial activity against Gram-positive bacteria, which is crucial for developing new antimicrobial agents .
Antiproliferative Activity
A study evaluated the antiproliferative effects of N-Methoxy-N-methylcinnamamide on several cancer cell lines, including MCF-7 (breast cancer) and HCT116 (colon cancer). The results are summarized in Table 1.
These findings indicate that N-Methoxy-N-methylcinnamamide has a potent antiproliferative effect on these cell lines, comparable to established chemotherapeutic agents.
Antioxidant Activity
The antioxidant capacity of N-Methoxy-N-methylcinnamamide was assessed using various assays, revealing its ability to scavenge free radicals effectively. The results are shown in Table 2.
These results suggest that the compound could be a valuable candidate for further development as an antioxidant agent.
Antibacterial Activity
N-Methoxy-N-methylcinnamamide exhibited notable antibacterial activity against various strains of bacteria. The minimum inhibitory concentration (MIC) values are presented in Table 3.
This antibacterial profile highlights its potential as a lead compound for developing new antibiotics.
Case Studies
Several case studies have explored the biological implications of N-Methoxy-N-methylcinnamamide:
- In Vivo Studies : In a recent study involving animal models, N-Methoxy-N-methylcinnamamide was administered to evaluate its anticancer effects. Results showed a significant reduction in tumor size compared to control groups.
- Mechanism of Action : Molecular docking studies revealed that N-Methoxy-N-methylcinnamamide binds effectively to active sites of enzymes involved in inflammation and cancer progression, such as iNOS and COX-2, suggesting a dual mechanism involving both anti-inflammatory and antiproliferative actions .
属性
IUPAC Name |
(E)-N-methoxy-N-methyl-3-phenylprop-2-enamide | |
---|---|---|
Source | PubChem | |
URL | https://pubchem.ncbi.nlm.nih.gov | |
Description | Data deposited in or computed by PubChem | |
InChI |
InChI=1S/C11H13NO2/c1-12(14-2)11(13)9-8-10-6-4-3-5-7-10/h3-9H,1-2H3/b9-8+ | |
Source | PubChem | |
URL | https://pubchem.ncbi.nlm.nih.gov | |
Description | Data deposited in or computed by PubChem | |
InChI Key |
PUWLTCZZUOAVPD-CMDGGOBGSA-N | |
Source | PubChem | |
URL | https://pubchem.ncbi.nlm.nih.gov | |
Description | Data deposited in or computed by PubChem | |
Canonical SMILES |
CN(C(=O)C=CC1=CC=CC=C1)OC | |
Source | PubChem | |
URL | https://pubchem.ncbi.nlm.nih.gov | |
Description | Data deposited in or computed by PubChem | |
Isomeric SMILES |
CN(C(=O)/C=C/C1=CC=CC=C1)OC | |
Source | PubChem | |
URL | https://pubchem.ncbi.nlm.nih.gov | |
Description | Data deposited in or computed by PubChem | |
Molecular Formula |
C11H13NO2 | |
Source | PubChem | |
URL | https://pubchem.ncbi.nlm.nih.gov | |
Description | Data deposited in or computed by PubChem | |
Molecular Weight |
191.23 g/mol | |
Source | PubChem | |
URL | https://pubchem.ncbi.nlm.nih.gov | |
Description | Data deposited in or computed by PubChem | |
Retrosynthesis Analysis
AI-Powered Synthesis Planning: Our tool employs the Template_relevance Pistachio, Template_relevance Bkms_metabolic, Template_relevance Pistachio_ringbreaker, Template_relevance Reaxys, Template_relevance Reaxys_biocatalysis model, leveraging a vast database of chemical reactions to predict feasible synthetic routes.
One-Step Synthesis Focus: Specifically designed for one-step synthesis, it provides concise and direct routes for your target compounds, streamlining the synthesis process.
Accurate Predictions: Utilizing the extensive PISTACHIO, BKMS_METABOLIC, PISTACHIO_RINGBREAKER, REAXYS, REAXYS_BIOCATALYSIS database, our tool offers high-accuracy predictions, reflecting the latest in chemical research and data.
Strategy Settings
Precursor scoring | Relevance Heuristic |
---|---|
Min. plausibility | 0.01 |
Model | Template_relevance |
Template Set | Pistachio/Bkms_metabolic/Pistachio_ringbreaker/Reaxys/Reaxys_biocatalysis |
Top-N result to add to graph | 6 |
Feasible Synthetic Routes
Q1: What is significant about the use of ionic liquids and microwave irradiation in the synthesis of aryl Weinreb amides like N-Methoxy-N-methylcinnamamide?
A1: The research highlights the advantages of using ionic liquids as solvents and microwave irradiation as a heating method for synthesizing aryl Weinreb amides like N-Methoxy-N-methylcinnamamide []. This combination offers several benefits over traditional methods:
体外研究产品的免责声明和信息
请注意,BenchChem 上展示的所有文章和产品信息仅供信息参考。 BenchChem 上可购买的产品专为体外研究设计,这些研究在生物体外进行。体外研究,源自拉丁语 "in glass",涉及在受控实验室环境中使用细胞或组织进行的实验。重要的是要注意,这些产品没有被归类为药物或药品,他们没有得到 FDA 的批准,用于预防、治疗或治愈任何医疗状况、疾病或疾病。我们必须强调,将这些产品以任何形式引入人类或动物的身体都是法律严格禁止的。遵守这些指南对确保研究和实验的法律和道德标准的符合性至关重要。