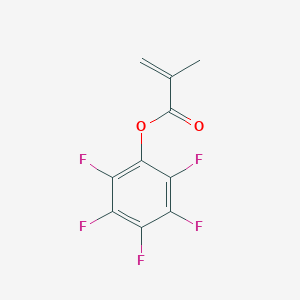
五氟苯基甲基丙烯酸酯
描述
Pentafluorophenyl methacrylate is an organic compound with the chemical formula C10H5F5O2. It is a colorless to pale yellow liquid with a strong, pungent odor. This compound is primarily used as a monomer in the synthesis of polymers and copolymers, particularly in the field of polymer chemistry. Pentafluorophenyl methacrylate is known for its high reactivity due to the presence of the pentafluorophenyl group, which makes it an excellent candidate for various chemical modifications and applications.
科学研究应用
Pentafluorophenyl methacrylate has a wide range of applications in scientific research, including:
Polymer Chemistry: It is used as a monomer in the synthesis of functional polymers and copolymers with unique properties, such as high thermal stability and chemical resistance.
Bioconjugation: The pentafluorophenyl group is highly reactive towards nucleophiles, making it useful for the conjugation of biomolecules, such as proteins and peptides, to synthetic polymers.
Material Science: Pentafluorophenyl methacrylate-based polymers are used in the development of advanced materials, such as coatings, adhesives, and nanocomposites.
Biomedical Applications: Functionalized polymers derived from pentafluorophenyl methacrylate are explored for drug delivery, tissue engineering, and diagnostic applications.
作用机制
Target of Action
Pentafluorophenyl methacrylate (PFMA) primarily targets the formation of polymers. The PFP unit in PFMA acts as an activated ester for coupling reactions, such as esterification and amidation . This compound is also a monomer for low refractive index polymers .
Mode of Action
PFMA interacts with its targets through a process known as anionic polymerization . This process involves the synthesis and polymerization of fluorine-substituted phenyl methacrylates . PFMA also facilitates the functionalization of single-chain polymer nanoparticles (SCNPs) without altering its backbone structure . The compound is prepared through intramolecular thiol-Michael addition crosslinking of thiol-functional precursor copolymers .
Biochemical Pathways
The primary biochemical pathway affected by PFMA is the polymerization pathway. The compound is involved in the synthesis and anionic polymerization of fluorine-substituted phenyl methacrylates . The resulting polymers are syndiotactically rich in structure .
Result of Action
The primary result of PFMA’s action is the formation of polymers. These polymers have a low refractive index and are syndiotactically rich in structure . PFMA also enables the functionalization of SCNPs, leading to the production of water-soluble SCNPs with fluorescent labels, ‘click’ functionality, amino acids, and even peptides .
Action Environment
The action of PFMA can be influenced by various environmental factors. For instance, the polymerization conditions, such as temperature and monomer concentration, can affect the yield and structure of the resulting polymers . Additionally, the presence of other compounds in the system can influence the functionalization of SCNPs .
生化分析
Biochemical Properties
Pentafluorophenyl Methacrylate is often used in the synthesis of polymers, primarily in photosensitive polymers, photocurable resins, and coatings . It can be added as a monomer to polymer systems, forming polymers through polymerization reactions . The Pentafluorophenyl (PFP) unit acts as an activated ester for coupling (esterification/amidation) reactions .
Cellular Effects
It is known that the compound can be used to create single-chain polymer nanoparticles (SCNPs), which can mimic the characteristics of proteins . These SCNPs can potentially influence cell function by enabling controlled delivery of therapeutics or imaging agents .
Molecular Mechanism
The molecular mechanism of Pentafluorophenyl Methacrylate primarily involves its role as a monomer in polymer synthesis . It participates in polymerization reactions to form polymers . Additionally, the PFP unit in the compound acts as an activated ester, facilitating coupling reactions .
准备方法
Synthetic Routes and Reaction Conditions
Pentafluorophenyl methacrylate is typically synthesized through the esterification of pentafluorophenol with methacrylic acid or its derivatives. The reaction is usually carried out in the presence of a catalyst, such as a strong acid (e.g., sulfuric acid) or a base (e.g., triethylamine), under controlled temperature and pressure conditions. The reaction can be represented as follows:
C6F5OH+CH2=C(CH3)COOH→C6F5OCOC(CH3)=CH2+H2O
Industrial Production Methods
In industrial settings, the production of pentafluorophenyl methacrylate involves large-scale esterification processes. The reaction is typically carried out in a continuous flow reactor to ensure consistent product quality and yield. The use of advanced catalysts and optimized reaction conditions, such as temperature, pressure, and reactant concentrations, is crucial for maximizing the efficiency of the process. Additionally, purification steps, such as distillation and recrystallization, are employed to obtain high-purity pentafluorophenyl methacrylate.
化学反应分析
Types of Reactions
Pentafluorophenyl methacrylate undergoes various chemical reactions, including:
Substitution Reactions: The pentafluorophenyl group can be substituted with nucleophiles, such as amines and alcohols, leading to the formation of new functionalized derivatives.
Polymerization Reactions: Pentafluorophenyl methacrylate can undergo free radical polymerization to form homopolymers and copolymers with other monomers.
Hydrolysis: The ester bond in pentafluorophenyl methacrylate can be hydrolyzed under acidic or basic conditions to yield pentafluorophenol and methacrylic acid.
Common Reagents and Conditions
Substitution Reactions: Common reagents include primary and secondary amines, alcohols, and thiols. These reactions are typically carried out in the presence of a base, such as triethylamine, at room temperature or slightly elevated temperatures.
Polymerization Reactions: Initiators such as azobisisobutyronitrile (AIBN) or benzoyl peroxide are used to initiate free radical polymerization. The reactions are conducted at temperatures ranging from 60°C to 80°C.
Hydrolysis: Acidic hydrolysis is performed using hydrochloric acid or sulfuric acid, while basic hydrolysis involves the use of sodium hydroxide or potassium hydroxide.
Major Products Formed
Substitution Reactions: Functionalized derivatives, such as pentafluorophenyl amides and esters.
Polymerization Reactions: Homopolymers and copolymers with varying properties depending on the comonomers used.
Hydrolysis: Pentafluorophenol and methacrylic acid.
相似化合物的比较
Pentafluorophenyl methacrylate can be compared with other methacrylate derivatives, such as:
Methacrylic Acid: Unlike pentafluorophenyl methacrylate, methacrylic acid lacks the electron-withdrawing pentafluorophenyl group, resulting in lower reactivity.
Methyl Methacrylate: Methyl methacrylate is a commonly used monomer in polymer synthesis, but it does not possess the unique reactivity and functionalization potential of pentafluorophenyl methacrylate.
Phenyl Methacrylate: Phenyl methacrylate has a phenyl group instead of a pentafluorophenyl group, leading to different reactivity and properties.
Pentafluorophenyl methacrylate stands out due to its high reactivity and versatility in chemical modifications, making it a valuable compound in various scientific and industrial applications.
属性
IUPAC Name |
(2,3,4,5,6-pentafluorophenyl) 2-methylprop-2-enoate | |
---|---|---|
Source | PubChem | |
URL | https://pubchem.ncbi.nlm.nih.gov | |
Description | Data deposited in or computed by PubChem | |
InChI |
InChI=1S/C10H5F5O2/c1-3(2)10(16)17-9-7(14)5(12)4(11)6(13)8(9)15/h1H2,2H3 | |
Source | PubChem | |
URL | https://pubchem.ncbi.nlm.nih.gov | |
Description | Data deposited in or computed by PubChem | |
InChI Key |
NIJWSVFNELSKMF-UHFFFAOYSA-N | |
Source | PubChem | |
URL | https://pubchem.ncbi.nlm.nih.gov | |
Description | Data deposited in or computed by PubChem | |
Canonical SMILES |
CC(=C)C(=O)OC1=C(C(=C(C(=C1F)F)F)F)F | |
Source | PubChem | |
URL | https://pubchem.ncbi.nlm.nih.gov | |
Description | Data deposited in or computed by PubChem | |
Molecular Formula |
C10H5F5O2 | |
Source | PubChem | |
URL | https://pubchem.ncbi.nlm.nih.gov | |
Description | Data deposited in or computed by PubChem | |
Related CAS |
111886-03-4 | |
Record name | 2-Propenoic acid, 2-methyl-, 2,3,4,5,6-pentafluorophenyl ester, homopolymer | |
Source | CAS Common Chemistry | |
URL | https://commonchemistry.cas.org/detail?cas_rn=111886-03-4 | |
Description | CAS Common Chemistry is an open community resource for accessing chemical information. Nearly 500,000 chemical substances from CAS REGISTRY cover areas of community interest, including common and frequently regulated chemicals, and those relevant to high school and undergraduate chemistry classes. This chemical information, curated by our expert scientists, is provided in alignment with our mission as a division of the American Chemical Society. | |
Explanation | The data from CAS Common Chemistry is provided under a CC-BY-NC 4.0 license, unless otherwise stated. | |
DSSTOX Substance ID |
DTXSID00159784 | |
Record name | Pentafluorophenylmethacrylate | |
Source | EPA DSSTox | |
URL | https://comptox.epa.gov/dashboard/DTXSID00159784 | |
Description | DSSTox provides a high quality public chemistry resource for supporting improved predictive toxicology. | |
Molecular Weight |
252.14 g/mol | |
Source | PubChem | |
URL | https://pubchem.ncbi.nlm.nih.gov | |
Description | Data deposited in or computed by PubChem | |
CAS No. |
13642-97-2 | |
Record name | Pentafluorophenylmethacrylate | |
Source | ChemIDplus | |
URL | https://pubchem.ncbi.nlm.nih.gov/substance/?source=chemidplus&sourceid=0013642972 | |
Description | ChemIDplus is a free, web search system that provides access to the structure and nomenclature authority files used for the identification of chemical substances cited in National Library of Medicine (NLM) databases, including the TOXNET system. | |
Record name | Pentafluorophenylmethacrylate | |
Source | EPA DSSTox | |
URL | https://comptox.epa.gov/dashboard/DTXSID00159784 | |
Description | DSSTox provides a high quality public chemistry resource for supporting improved predictive toxicology. | |
Record name | Pentafluorophenyl Methacrylate | |
Source | European Chemicals Agency (ECHA) | |
URL | https://echa.europa.eu/information-on-chemicals | |
Description | The European Chemicals Agency (ECHA) is an agency of the European Union which is the driving force among regulatory authorities in implementing the EU's groundbreaking chemicals legislation for the benefit of human health and the environment as well as for innovation and competitiveness. | |
Explanation | Use of the information, documents and data from the ECHA website is subject to the terms and conditions of this Legal Notice, and subject to other binding limitations provided for under applicable law, the information, documents and data made available on the ECHA website may be reproduced, distributed and/or used, totally or in part, for non-commercial purposes provided that ECHA is acknowledged as the source: "Source: European Chemicals Agency, http://echa.europa.eu/". Such acknowledgement must be included in each copy of the material. ECHA permits and encourages organisations and individuals to create links to the ECHA website under the following cumulative conditions: Links can only be made to webpages that provide a link to the Legal Notice page. | |
Retrosynthesis Analysis
AI-Powered Synthesis Planning: Our tool employs the Template_relevance Pistachio, Template_relevance Bkms_metabolic, Template_relevance Pistachio_ringbreaker, Template_relevance Reaxys, Template_relevance Reaxys_biocatalysis model, leveraging a vast database of chemical reactions to predict feasible synthetic routes.
One-Step Synthesis Focus: Specifically designed for one-step synthesis, it provides concise and direct routes for your target compounds, streamlining the synthesis process.
Accurate Predictions: Utilizing the extensive PISTACHIO, BKMS_METABOLIC, PISTACHIO_RINGBREAKER, REAXYS, REAXYS_BIOCATALYSIS database, our tool offers high-accuracy predictions, reflecting the latest in chemical research and data.
Strategy Settings
Precursor scoring | Relevance Heuristic |
---|---|
Min. plausibility | 0.01 |
Model | Template_relevance |
Template Set | Pistachio/Bkms_metabolic/Pistachio_ringbreaker/Reaxys/Reaxys_biocatalysis |
Top-N result to add to graph | 6 |
Feasible Synthetic Routes
Q1: What is the molecular formula and weight of PFPMA?
A1: The molecular formula of PFPMA is C10H5F5O2, and its molecular weight is 252.14 g/mol.
Q2: What are the key spectroscopic characteristics of PFPMA?
A2: PFPMA can be characterized using various spectroscopic techniques, including:
- 1H and 19F NMR Spectroscopy: Provides valuable information about the structure, composition, and purity of PFPMA and its polymers. [, , , , , , , , , , ]
- FTIR Spectroscopy: Useful for identifying functional groups and monitoring chemical modifications, such as the conversion of the pentafluorophenyl ester group during post-polymerization reactions. [, , , , ]
- XPS: Provides information about the elemental composition and chemical states of elements present in PFPMA-containing materials, particularly thin films. [, ]
Q3: How does the incorporation of PFPMA affect the glass transition temperature (Tg) of copolymers?
A3: Incorporating PFPMA into copolymers, such as those with methyl methacrylate (MMA), generally leads to an increase in Tg. This increase can exhibit positive deviation from the Gordon-Taylor equation, indicating interactions between PFPMA and the comonomer units. [, , ]
Q4: What is the impact of PFPMA on the water absorption properties of polymers?
A4: Adding PFPMA to polymers like PMMA can significantly reduce their water absorption. For instance, incorporating 20 wt% PFPMA into PMMA decreased water absorption to 0.4 wt%, compared to 1.8 wt% for pure PMMA. []
Q5: How is PFPMA utilized in post-polymerization modification strategies?
A5: PFPMA contains highly reactive pentafluorophenyl ester groups that readily undergo nucleophilic substitution reactions with amines, thiols, and other nucleophiles. This reactivity makes PFPMA-containing polymers ideal platforms for post-polymerization modification, enabling the introduction of a wide range of functionalities. [, , , , , , , , , , , , , , , , , ]
Q6: Can you provide specific examples of post-polymerization modifications using PFPMA-based polymers?
A6: Several examples highlight the versatility of PFPMA in post-polymerization modifications:
- Amine Modification: Reacting PFPMA polymers with amines, such as diaminohexane or amino-containing peptides, allows the introduction of various functional groups, including amines, peptides, and fluorescent dyes. [, , , , , , , , , , , , , ]
- Thiol-Ene Reactions: PFPMA can be copolymerized with monomers containing alkene groups, enabling subsequent thiol-ene reactions for further functionalization. [, ]
- Click Chemistry: PFPMA polymers with alkyne functionalities can be modified using copper-catalyzed azide-alkyne cycloaddition (CuAAC) reactions to introduce a variety of functional groups. [, ]
Q7: How does the presence of fluorine atoms in PFPMA contribute to its reactivity?
A7: The electron-withdrawing nature of fluorine atoms in the pentafluorophenyl group significantly enhances the reactivity of the ester group towards nucleophilic attack, making PFPMA a highly sought-after monomer for post-polymerization modification strategies. [, , , , , , , ]
Q8: What makes PFPMA suitable for developing drug delivery systems?
A8: The reactive ester groups in PFPMA-based polymers enable the conjugation of therapeutic molecules, like peptides and drugs, to create polymer-drug conjugates. These conjugates can enhance drug solubility, stability, and targeted delivery to specific cells or tissues. [, , , , , , , , ]
Q9: How is PFPMA used in the fabrication of nanomaterials?
A9: PFPMA-based block copolymers can self-assemble into various nanostructures, including micelles, vesicles (polymersomes), and nanogels, in solution. These nanomaterials hold potential applications in drug delivery, imaging, and sensing. [, , , , , , ]
Q10: Can you provide examples of specific applications of PFPMA-based nanomaterials in drug delivery?
A10: PFPMA-based nanomaterials show promise in several drug delivery applications:
- siRNA Delivery: Cationic nanohydrogel particles synthesized from PFPMA-containing block copolymers effectively complex and transport siRNA into cells. [, ]
- Controlled Drug Release: PFPMA-based polymersomes encapsulate both hydrophilic and hydrophobic drugs, enabling controlled release profiles for targeted therapy. [, , ]
- Stimuli-Responsive Nanogels: Incorporating stimuli-responsive moieties into PFPMA-based nanogels allows for triggered drug release in response to specific environmental cues, such as pH or redox potential. []
Q11: What is known about the biocompatibility of PFPMA-based materials?
A11: While PFPMA itself is not inherently biocompatible, post-polymerization modification with biocompatible molecules like poly(ethylene glycol) (PEG) can significantly improve the biocompatibility of PFPMA-containing materials. [, , ]
Q12: Are there biodegradable PFPMA-based polymers?
A12: Research has explored the synthesis of biodegradable PFPMA-based polymers by incorporating cleavable linkers or degradable segments into the polymer backbone, potentially enabling controlled degradation and elimination from the body. []
体外研究产品的免责声明和信息
请注意,BenchChem 上展示的所有文章和产品信息仅供信息参考。 BenchChem 上可购买的产品专为体外研究设计,这些研究在生物体外进行。体外研究,源自拉丁语 "in glass",涉及在受控实验室环境中使用细胞或组织进行的实验。重要的是要注意,这些产品没有被归类为药物或药品,他们没有得到 FDA 的批准,用于预防、治疗或治愈任何医疗状况、疾病或疾病。我们必须强调,将这些产品以任何形式引入人类或动物的身体都是法律严格禁止的。遵守这些指南对确保研究和实验的法律和道德标准的符合性至关重要。