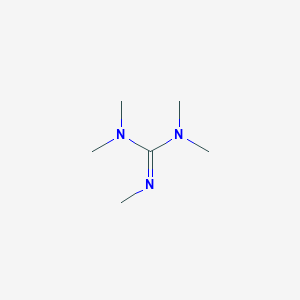
1,1,2,3,3-pentamethylguanidine
概述
描述
1,1,2,3,3-Pentamethylguanidine (PMG; CAS 13439-84-4) is a substituted guanidine derivative with the molecular formula C₆H₁₅N₃ and a molecular weight of 129.207 g/mol . It is characterized by five methyl groups attached to the guanidine core, resulting in a sterically hindered structure. PMG exhibits a boiling point of 155–160°C, a predicted density of 0.85 g/cm³, and a pKa of 13.78, indicating strong basicity . Key applications include its use as a catalyst in organic synthesis and as a functionalizing agent in anion exchange membranes (AEMs), where it enhances ionic conductivity (3.12 × 10⁻² S·cm⁻¹) and thermal stability (up to 280°C) .
准备方法
Synthetic Routes and Reaction Conditions:
Reaction with Formaldehyde and Formic Acid: One common method involves the reaction of diethylenetriamine with formaldehyde and formic acid. This reaction typically yields around 70% of the desired product.
Catalytic Hydrogenation: Another method involves the reaction of diethylenetriamine with formaldehyde in the presence of a catalyst and hydrogen gas.
Industrial Production Methods: The industrial production of N,N,N’,N’,N’'-Pentamethylguanidine typically follows the catalytic hydrogenation method due to its higher yield and efficiency. The process involves careful control of reaction conditions to ensure high purity and yield of the final product.
化学反应分析
Types of Reactions:
Oxidation: N,N,N’,N’,N’'-Pentamethylguanidine can undergo oxidation reactions, often resulting in the formation of corresponding oxides.
Substitution: This compound can participate in substitution reactions, particularly with electrophiles, due to its strong basicity.
Complex Formation: It can form complexes with transition metals, which are utilized in various catalytic processes.
Common Reagents and Conditions:
Oxidizing Agents: Common oxidizing agents include hydrogen peroxide and potassium permanganate.
Electrophiles: Typical electrophiles include alkyl halides and acyl chlorides.
Catalysts: Transition metal catalysts such as copper bromide are often used in complex formation reactions.
Major Products:
Oxides: Oxidation reactions typically yield oxides of the compound.
Substituted Products: Substitution reactions result in various substituted derivatives depending on the electrophile used.
Metal Complexes: Complex formation reactions yield metal complexes that are useful in catalysis.
科学研究应用
Chemical Properties and Mechanism of Action
PMG is characterized by its strong basicity and ability to form stable complexes with transition metals. These properties enable it to act as a catalyst in various chemical reactions. Its mechanism of action primarily involves:
- Deprotonation : PMG can deprotonate substrates, facilitating nucleophilic substitution and elimination reactions.
- Complex Formation : It interacts with transition metals to form stable complexes that catalyze polymerization and other reactions.
Catalysis
PMG is widely utilized as a catalyst in polymerization reactions. Notable applications include:
- Thermoresponsive Polymer Synthesis : PMG facilitates the synthesis of polymers that respond to temperature changes, making it valuable in developing smart materials.
- Transesterification Reactions : PMG-based polycationic systems have shown remarkable efficiency in catalyzing the transesterification of triglycerides, achieving up to 100% conversion rates within short reaction times .
Biological Applications
In the field of medicinal chemistry, PMG has been investigated for its potential in drug delivery systems:
- Metal Complexes for Drug Delivery : The ability of PMG to form complexes with metals enhances its application in designing drug delivery systems that can respond to environmental stimuli such as temperature.
Fuel Cell Technology
PMG has been explored in the development of anion exchange membranes for alkaline fuel cells:
- Stability Assessment : Studies have focused on synthesizing guanidinium-based anion exchange membranes using PMG and assessing their stability under operational conditions .
Case Study 1: Transesterification Catalysis
A study evaluated the efficiency of PMG-based poly(hexamethylene biguanide) (PHMBG) in catalyzing the transesterification of sunflower oil with methanol. The results indicated:
- Conversion Rates : PHMBG achieved 80–100% triglyceride conversion within 0.5 hours at 70°C.
- Comparison with Other Catalysts : PEI-based networks catalyzed the reaction at rates 8-12 times slower than PHMBG-based systems .
Case Study 2: Drug Delivery Systems
Research on PMG's role in drug delivery systems highlighted:
- Temperature-Responsive Behavior : PMG's metal complexes demonstrated significant potential for controlled drug release in response to temperature variations, which could enhance therapeutic efficacy.
Table 1: Comparison of Catalytic Efficiency
Catalyst Type | Reaction Type | Conversion Rate (%) | Time (hours) |
---|---|---|---|
PMG-based PHMBG | Transesterification | 80–100 | 0.5 |
PEI-based Networks | Transesterification | Slower (8-12x) | - |
PMG Metal Complexes | Drug Delivery | Variable | - |
Table 2: Applications in Fuel Cell Technology
Application Area | Description | Stability Assessment |
---|---|---|
Anion Exchange Membranes | Use of PMG for synthesizing membranes for fuel cells | Ongoing research |
作用机制
Mechanism: N,N,N’,N’,N’'-Pentamethylguanidine exerts its effects primarily through its strong basicity. It can deprotonate various substrates, facilitating nucleophilic substitution and elimination reactions. Additionally, its ability to form stable complexes with transition metals allows it to act as a catalyst in various polymerization and other chemical reactions .
Molecular Targets and Pathways:
Deprotonation: Targets acidic protons in substrates, facilitating various chemical transformations.
Complex Formation: Interacts with transition metals to form stable complexes that catalyze polymerization and other reactions.
相似化合物的比较
Comparison with Similar Guanidine Derivatives
Structural and Molecular Properties
The following table compares PMG with structurally related guanidine compounds:
Compound | Molecular Formula | Molecular Weight (g/mol) | CAS Number | Substituent Pattern |
---|---|---|---|---|
1,1,2,3,3-Pentamethylguanidine (PMG) | C₆H₁₅N₃ | 129.207 | 13439-84-4 | N¹,N¹,N²,N³,N³-pentamethyl |
1,1,3,3-Tetramethylguanidine (TMG) | C₅H₁₃N₃ | 115.18 | 80-70-6 | N¹,N¹,N³,N³-tetramethyl |
1,2,3-Tricyclohexylguanidine (TCG) | C₂₁H₃₆N₃ | 330.53 | N/A | N¹,N²,N³-tricyclohexyl |
1,3-Dicyclohexyl-2-n-octylguanidine (DCOG) | C₂₀H₃₉N₃ | 321.55 | N/A | N¹,N³-dicyclohexyl; N²-n-octyl |
Key Observations :
- Cyclohexyl-substituted derivatives (TCG, DCOG) exhibit higher molecular weights and enhanced symmetry, which improve catalytic activity in transesterification reactions .
Physicochemical and Catalytic Properties
Compound | Boiling Point (°C) | Density (g/cm³) | pKa | Catalytic Activity (Transesterification) |
---|---|---|---|---|
PMG | 155–160 | 0.85 | 13.78 | Moderate |
TMG | 52–54 (at 11 mmHg) | 0.918 | ~13.5* | High (non-nucleophilic base) |
TCG | N/A | N/A | >14 | Very High |
DCOG | N/A | N/A | >14 | High |
Note: TMG’s pKa is inferred from its role as a strong base comparable to DBU/DBN .
Catalytic Performance: In the transesterification of soybean oil with methanol, the activity order is TBD > TCG > DCOG > MTBD > PMG, correlating with base strength and structural symmetry. PMG’s lower activity is attributed to its acyclic, asymmetric substituents compared to TCG’s cyclic symmetry .
生物活性
1,1,2,3,3-Pentamethylguanidine (PMG) is a guanidine derivative known for its unique chemical properties and significant biological activities. This compound has garnered attention in various fields, including medicinal chemistry and materials science. This article reviews the biological activity of PMG, focusing on its mechanisms of action, applications in research, and relevant case studies.
- Molecular Formula : C6H15N3
- Molecular Weight : 129.207 g/mol
- CAS Number : 13439-84-4
PMG is characterized by its strong basicity and ability to act as a nucleophile due to the presence of multiple methyl groups that enhance its stability and reactivity in various chemical environments .
- Enzyme Inhibition : PMG has been shown to inhibit various enzymes, including Rho-associated protein kinases (ROCK1 and ROCK2). These kinases play crucial roles in cellular processes such as cytoskeletal organization and cell migration. Inhibition of ROCK2 has been linked to antifibrotic effects, while ROCK1 inhibition offers potential therapeutic benefits in conditions like hypertension and cancer .
- Antimicrobial Activity : PMG exhibits antimicrobial properties against a range of pathogens. Its mechanism involves disrupting microbial cell membranes and inhibiting essential cellular functions. This property makes PMG a candidate for developing new antimicrobial agents .
- Polymeric Applications : In polymer chemistry, PMG is used to synthesize guanidinium-based anion exchange membranes. These membranes have shown promise in applications such as fuel cells and water purification systems due to their enhanced ionic conductivity and stability .
1. ROCK Kinase Inhibition
A study evaluated the effects of PMG on ROCK1 and ROCK2 activity through kinase assays. The results indicated that PMG selectively inhibited ROCK2 with an IC50 value significantly lower than that for ROCK1, suggesting its potential use in treating fibrotic diseases .
2. Antimicrobial Efficacy
Research conducted on the antimicrobial activity of PMG revealed that it effectively inhibited the growth of several bacterial strains, including Escherichia coli and Staphylococcus aureus. The minimum inhibitory concentration (MIC) was determined to be 32 µg/mL for E. coli, highlighting its potential as a therapeutic agent against resistant bacterial infections .
3. Polymer Synthesis
In another study, PMG was utilized in the synthesis of polymeric guanidine derivatives. The resulting materials demonstrated enhanced mechanical properties and thermal stability compared to traditional polymers. These advancements suggest potential applications in biomedical devices and coatings .
Data Table: Biological Activities of this compound
常见问题
Basic Research Questions
Q. What are the recommended synthetic routes for 1,1,2,3,3-pentamethylguanidine, and how do reaction conditions influence yield?
- Methodology : The compound can be synthesized via alkylation of guanidine derivatives. For example, methylating agents like methyl iodide or dimethyl sulfate in a polar aprotic solvent (e.g., DMF) under inert conditions. Temperature control (40–60°C) and stoichiometric ratios of methylating agents are critical to avoid over-alkylation. Yield optimization requires monitoring by TLC or HPLC, with purification via fractional distillation or recrystallization .
- Data Contradictions : Conflicting reports on optimal solvent (DMF vs. THF) may arise due to differences in reaction scale or catalyst use. Comparative studies using -NMR to track intermediate formation are advised to resolve discrepancies .
Q. How can researchers characterize the purity and structural integrity of this compound?
- Methodology : Use a combination of spectroscopic techniques:
- -NMR : Peaks at δ 2.8–3.2 ppm (N–CH groups) and δ 1.2–1.5 ppm (C–CH).
- LC-MS : Confirm molecular ion peak at m/z 129.2 (M+H).
- Elemental Analysis : Validate %C, %H, and %N against theoretical values (C: 55.78%, H: 11.70%, N: 32.52%) .
- Pitfalls : Impurities like residual solvents or unreacted intermediates may skew results. Use preparative HPLC with a C18 column for high-purity isolation .
Advanced Research Questions
Q. What is the coordination behavior of this compound with transition metals, and how does it compare to other guanidine derivatives?
- Methodology : Study its ligand properties using Pt(II) or Pd(II) complexes. For example, react with [Pt(trpy)Cl] (trpy = terpyridine) in acetonitrile. Characterize via X-ray crystallography to confirm bonding modes (terminal vs. bridging). Compare stability constants (log K) with 1,1,3,3-tetramethylguanidine using UV-Vis titration .
- Key Findings : Pentamethylguanidine’s steric bulk may reduce coordination efficiency but enhance complex solubility in nonpolar solvents. Contrasts with smaller ligands like unsubstituted guanidine, which form stronger but less stable bonds .
Q. How does this compound interact with biomolecules, such as nucleic acids or enzymes?
- Methodology : Investigate its role as a chaotropic agent in DNA/RNA extraction protocols. Compare denaturation efficiency with guanidine thiocyanate (GTC) using fluorescence-based assays (e.g., SYBR Green binding). For enzymatic studies, test inhibition of Taq polymerase via competitive binding assays .
- Contradictions : While GTC is more potent in RNA stabilization, pentamethylguanidine’s lower toxicity may favor in vivo applications. Resolve discrepancies by measuring RNase inhibition at varying concentrations .
Q. What are the metabolic pathways and environmental persistence of this compound?
- Methodology : Use -labeled compound to track degradation in microbial cultures (e.g., Synechococcus spp.). Analyze metabolites via LC-MS/MS. Compare degradation rates with unmethylated guanidine under aerobic/anaerobic conditions .
- Advanced Insight : Engineered cyanobacteria expressing Sll1077 (guanidine-degrading enzyme) show partial degradation. Directed evolution of this enzyme could enhance bioremediation efficiency .
Q. Methodological Guidance for Research Design
Q. How to address batch-to-batch variability in synthetic yields or bioactivity assays?
- Solution : Implement strict QC protocols:
- Synthesis : Standardize reaction time, temperature, and purification steps.
- Bioassays : Include internal controls (e.g., commercial guanidine HCl) and request peptide content analysis if used in sensitive assays .
Q. What computational tools are suitable for modeling interactions of this compound?
- Approach : Perform DFT calculations (e.g., Gaussian 09) to optimize geometry and predict binding energies with metal ions. Molecular dynamics (MD) simulations (e.g., GROMACS) can model enzyme interactions, as demonstrated for phosphoryl guanidine oligonucleotides .
属性
IUPAC Name |
1,1,2,3,3-pentamethylguanidine | |
---|---|---|
Source | PubChem | |
URL | https://pubchem.ncbi.nlm.nih.gov | |
Description | Data deposited in or computed by PubChem | |
InChI |
InChI=1S/C6H15N3/c1-7-6(8(2)3)9(4)5/h1-5H3 | |
Source | PubChem | |
URL | https://pubchem.ncbi.nlm.nih.gov | |
Description | Data deposited in or computed by PubChem | |
InChI Key |
ISNICOKBNZOJQG-UHFFFAOYSA-N | |
Source | PubChem | |
URL | https://pubchem.ncbi.nlm.nih.gov | |
Description | Data deposited in or computed by PubChem | |
Canonical SMILES |
CN=C(N(C)C)N(C)C | |
Source | PubChem | |
URL | https://pubchem.ncbi.nlm.nih.gov | |
Description | Data deposited in or computed by PubChem | |
Molecular Formula |
C6H15N3 | |
Source | PubChem | |
URL | https://pubchem.ncbi.nlm.nih.gov | |
Description | Data deposited in or computed by PubChem | |
DSSTOX Substance ID |
DTXSID70158683 | |
Record name | N,N,N',N',N''-Pentamethylguanidine | |
Source | EPA DSSTox | |
URL | https://comptox.epa.gov/dashboard/DTXSID70158683 | |
Description | DSSTox provides a high quality public chemistry resource for supporting improved predictive toxicology. | |
Molecular Weight |
129.20 g/mol | |
Source | PubChem | |
URL | https://pubchem.ncbi.nlm.nih.gov | |
Description | Data deposited in or computed by PubChem | |
CAS No. |
13439-84-4 | |
Record name | N,N,N',N',N''-Pentamethylguanidine | |
Source | ChemIDplus | |
URL | https://pubchem.ncbi.nlm.nih.gov/substance/?source=chemidplus&sourceid=0013439844 | |
Description | ChemIDplus is a free, web search system that provides access to the structure and nomenclature authority files used for the identification of chemical substances cited in National Library of Medicine (NLM) databases, including the TOXNET system. | |
Record name | N,N,N',N',N''-Pentamethylguanidine | |
Source | EPA DSSTox | |
URL | https://comptox.epa.gov/dashboard/DTXSID70158683 | |
Description | DSSTox provides a high quality public chemistry resource for supporting improved predictive toxicology. | |
Synthesis routes and methods I
Procedure details
Synthesis routes and methods II
Procedure details
Retrosynthesis Analysis
AI-Powered Synthesis Planning: Our tool employs the Template_relevance Pistachio, Template_relevance Bkms_metabolic, Template_relevance Pistachio_ringbreaker, Template_relevance Reaxys, Template_relevance Reaxys_biocatalysis model, leveraging a vast database of chemical reactions to predict feasible synthetic routes.
One-Step Synthesis Focus: Specifically designed for one-step synthesis, it provides concise and direct routes for your target compounds, streamlining the synthesis process.
Accurate Predictions: Utilizing the extensive PISTACHIO, BKMS_METABOLIC, PISTACHIO_RINGBREAKER, REAXYS, REAXYS_BIOCATALYSIS database, our tool offers high-accuracy predictions, reflecting the latest in chemical research and data.
Strategy Settings
Precursor scoring | Relevance Heuristic |
---|---|
Min. plausibility | 0.01 |
Model | Template_relevance |
Template Set | Pistachio/Bkms_metabolic/Pistachio_ringbreaker/Reaxys/Reaxys_biocatalysis |
Top-N result to add to graph | 6 |
Feasible Synthetic Routes
体外研究产品的免责声明和信息
请注意,BenchChem 上展示的所有文章和产品信息仅供信息参考。 BenchChem 上可购买的产品专为体外研究设计,这些研究在生物体外进行。体外研究,源自拉丁语 "in glass",涉及在受控实验室环境中使用细胞或组织进行的实验。重要的是要注意,这些产品没有被归类为药物或药品,他们没有得到 FDA 的批准,用于预防、治疗或治愈任何医疗状况、疾病或疾病。我们必须强调,将这些产品以任何形式引入人类或动物的身体都是法律严格禁止的。遵守这些指南对确保研究和实验的法律和道德标准的符合性至关重要。