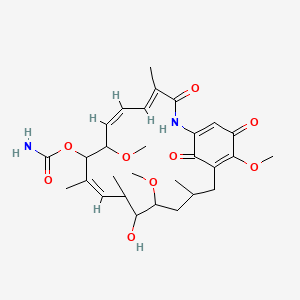
Geldanamycin
描述
Geldanamycin is a 1,4-benzoquinone ansamycin antitumor antibiotic that was originally discovered in the bacterium Streptomyces hygroscopicus . It is known for its ability to inhibit the function of Heat Shock Protein 90 (Hsp90) by binding to its unusual ADP/ATP-binding pocket . Hsp90 client proteins play crucial roles in the regulation of the cell cycle, cell growth, cell survival, apoptosis, angiogenesis, and oncogenesis .
科学研究应用
Geldanamycin has a wide range of scientific research applications, particularly in the fields of chemistry, biology, medicine, and industry:
Chemistry: this compound and its derivatives are studied for their unique chemical properties and potential as chemical probes.
Biology: In biological research, this compound is used to study the function of Hsp90 and its role in cellular processes.
Medicine: this compound has shown potential as an anti-cancer agent due to its ability to inhibit Hsp90, leading to the degradation of oncogenic proteins. its clinical use is limited by its hepatotoxicity.
作用机制
Target of Action
Geldanamycin primarily targets Heat Shock Protein 90 (Hsp90) . Hsp90 is a molecular chaperone that plays a crucial role in the regulation of various cell signaling proteins, including many tyrosine and serine/threonine kinases, which are involved in cell proliferation and survival .
Mode of Action
This compound binds to the unusual ADP/ATP-binding pocket of Hsp90, thereby inhibiting its function as a molecular chaperone . This interaction disrupts the chaperone’s function, leading to the destabilization of Hsp90 protein clients .
Biochemical Pathways
The inhibition of Hsp90 by this compound affects several oncogenic signaling pathways. It results in the downregulation of several Hsp90 protein clients and downstream effectors, such as membrane receptors (IGF-IR and c-Met), protein kinases (Akt, IKKα, IKKβ, and Erk1/2), and transcription factors (FOXOs and NF-κΒ) . This leads to the impairment of proliferative oncogenic signaling and reduction of cell motility .
Pharmacokinetics
It is known that this compound is distributed widely to tissues but is retained for longer in tumors than in normal tissues .
Result of Action
Upon this compound treatment, cells are prominently arrested in the G1 phase of the cell cycle and eventually undergo programmed cell death via combined activation of apoptosis and autophagy . Furthermore, this compound induces the degradation of proteins that are mutated or overexpressed in tumor cells such as v-Src, Bcr-Abl, p53, and ERBB2 .
Action Environment
The action of this compound can be influenced by various environmental factors. For instance, the presence of surfactant compounds, the concentration of viable spores in the inoculum, and shear can significantly affect the morphology of the filamentous organism Streptomyces hygroscopicus var. geldanus, which produces this compound . These factors can be exploited to limit pellet formation to an appropriate size, thereby maximizing this compound production .
生化分析
Biochemical Properties
Geldanamycin plays a crucial role in biochemical reactions by binding to the unusual ADP/ATP-binding pocket of Hsp90. This interaction inhibits the chaperone function of Hsp90, leading to the destabilization and degradation of its client proteins. These client proteins include various kinases, transcription factors, and other proteins involved in cell cycle regulation, cell growth, and survival . For example, this compound induces the degradation of proteins such as v-Src, Bcr-Abl, p53, and ERBB2, which are often mutated or overexpressed in tumor cells .
Cellular Effects
This compound has significant effects on various types of cells and cellular processes. By inhibiting Hsp90, this compound disrupts multiple oncogenic signaling pathways, leading to cell cycle arrest and programmed cell death (apoptosis) in cancer cells . It also affects cell signaling pathways, gene expression, and cellular metabolism. For instance, this compound treatment results in the downregulation of several Hsp90 client proteins and downstream effectors, such as membrane receptors (IGF-IR and c-Met), protein kinases (Akt, IKKα, IKKβ, and Erk1/2), and transcription factors (FOXOs and NF-κB), thereby impairing proliferative signaling and reducing cell motility .
Molecular Mechanism
At the molecular level, this compound exerts its effects by binding to the ADP/ATP-binding pocket of Hsp90, thereby inhibiting its chaperone activity . This inhibition prevents the proper folding and stabilization of Hsp90 client proteins, leading to their ubiquitination and subsequent proteasomal degradation . The disruption of Hsp90 function also affects the heat shock response and induces the expression of stress-related proteins, further contributing to the antitumor activity of this compound .
Temporal Effects in Laboratory Settings
In laboratory settings, the effects of this compound can change over time. This compound has been shown to induce cytotoxicity and enhance the radiation response of tumor cells within a temporal range of 6 to 48 hours after drug exposure . Additionally, this compound’s stability and degradation can influence its long-term effects on cellular function. For example, this compound treatment has been observed to induce apoptosis and autophagy in bladder cancer cells over time .
Dosage Effects in Animal Models
The effects of this compound vary with different dosages in animal models. In a study on mice with intracerebral hemorrhage, this compound at a high dose (10 mg/kg) significantly ameliorated brain water content and improved neurological outcomes . The therapeutic efficacy and potential toxic effects of this compound at different dosages need to be carefully evaluated to determine the optimal dosage for clinical use .
Metabolic Pathways
This compound is involved in various metabolic pathways, particularly those related to its biosynthesis and degradation. It is synthesized by a Type I polyketide synthase in Streptomyces hygroscopicus, involving several enzymatic steps such as hydroxylation, o-methylation, carbamoylation, and oxidation . This compound also interacts with metabolic pathways related to its antitumor activity, such as the inhibition of Hsp90 and the subsequent degradation of its client proteins .
Transport and Distribution
Within cells and tissues, this compound is transported and distributed through various mechanisms. It has been shown to alter endosomal sorting, diverting cargo destined for the recycling pathway into the lysosomal pathway . Additionally, this compound enhances the retrograde transport of certain toxins, such as Shiga toxin, to the Golgi apparatus . These transport and distribution mechanisms contribute to the localization and accumulation of this compound within specific cellular compartments.
Subcellular Localization
This compound primarily localizes to the cytoplasm, where it interacts with Hsp90 and its client proteins . The inhibition of Hsp90 by this compound affects the subcellular localization of various proteins, including steroid hormone receptors such as glucocorticoid receptors and progesterone receptors . By blocking the cytoplasmic retention of these receptors, this compound promotes their translocation to the nucleus, thereby influencing their activity and function .
准备方法
Synthetic Routes and Reaction Conditions: Geldanamycin is synthesized by a Type I polyketide synthase. The genes gelA, gelB, and gelC encode for the polyketide synthase . The polyketide synthase is first loaded with 3-amino-5-hydroxybenzoic acid (AHBA). It then utilizes malonyl-CoA, methylmalonyl-CoA, and methoxymalonyl-CoA to synthesize the precursor molecule Prothis compound . This precursor undergoes several enzymatic and non-enzymatic tailoring steps, including hydroxylation, o-methylation, carbamoylation, and oxidation, to produce the active molecule this compound .
Industrial Production Methods: Industrial production of this compound involves fermentation processes using Streptomyces hygroscopicus strains. The biosynthesis of this compound is sensitive to the inorganic phosphate concentration in the medium, which is regulated through the two-component system PhoR-PhoP .
化学反应分析
Types of Reactions: Geldanamycin undergoes various chemical reactions, including oxidation, reduction, and substitution reactions.
Common Reagents and Conditions:
Oxidation: this compound can be oxidized using reagents such as hydrogen peroxide or other oxidizing agents.
Reduction: Reduction reactions can be carried out using reducing agents like sodium borohydride.
Substitution: Substitution reactions often involve nucleophilic reagents that can replace functional groups on the this compound molecule.
Major Products: The major products formed from these reactions include various derivatives of this compound, which are often evaluated for their biological activity and potential therapeutic applications .
相似化合物的比较
- Herbimycin A
- Herbimycin B
- Herbimycin C
- Macbecin II
- 17-AAG (17-allylamino-17-demethoxygeldanamycin)
Uniqueness of this compound: this compound is unique due to its potent anti-cancer activity and its ability to specifically inhibit Hsp90. its clinical use is limited by its hepatotoxicity, which has led to the development of analogues with improved safety profiles .
属性
CAS 编号 |
30562-34-6 |
---|---|
分子式 |
C29H40N2O9 |
分子量 |
560.6 g/mol |
IUPAC 名称 |
[(4Z,6Z,8R,9R,10Z,12R,13S,14R,16R)-13-hydroxy-8,14,19-trimethoxy-4,10,12,16-tetramethyl-3,20,22-trioxo-2-azabicyclo[16.3.1]docosa-1(21),4,6,10,18-pentaen-9-yl] carbamate |
InChI |
InChI=1S/C29H40N2O9/c1-15-11-19-25(34)20(14-21(32)27(19)39-7)31-28(35)16(2)9-8-10-22(37-5)26(40-29(30)36)18(4)13-17(3)24(33)23(12-15)38-6/h8-10,13-15,17,22-24,26,33H,11-12H2,1-7H3,(H2,30,36)(H,31,35)/b10-8-,16-9-,18-13-/t15-,17-,22-,23-,24+,26-/m1/s1 |
InChI 键 |
QTQAWLPCGQOSGP-WKALPRSISA-N |
手性 SMILES |
C[C@H]1C[C@H]([C@H]([C@@H](/C=C(\[C@H]([C@@H](/C=C\C=C(/C(=O)NC2=CC(=O)C(=C(C1)C2=O)OC)\C)OC)OC(=O)N)/C)C)O)OC |
SMILES |
CC1CC(C(C(C=C(C(C(C=CC=C(C(=O)NC2=CC(=O)C(=C(C1)C2=O)OC)C)OC)OC(=O)N)C)C)O)OC |
规范 SMILES |
CC1CC(C(C(C=C(C(C(C=CC=C(C(=O)NC2=CC(=O)C(=C(C1)C2=O)OC)C)OC)OC(=O)N)C)C)O)OC |
外观 |
Solid powder |
30562-34-6 | |
纯度 |
>98% (or refer to the Certificate of Analysis) |
保质期 |
>5 years if stored properly |
溶解度 |
Soluble in DMSO, not in water |
储存 |
Dry, dark and at 0 - 4 C for short term (days to weeks) or -20 C for long term (months to years). |
同义词 |
NSC 122750; NSC-122750; NSC122750; U 29135; U-29135; U29135. Geldanamycin. |
产品来源 |
United States |
Retrosynthesis Analysis
AI-Powered Synthesis Planning: Our tool employs the Template_relevance Pistachio, Template_relevance Bkms_metabolic, Template_relevance Pistachio_ringbreaker, Template_relevance Reaxys, Template_relevance Reaxys_biocatalysis model, leveraging a vast database of chemical reactions to predict feasible synthetic routes.
One-Step Synthesis Focus: Specifically designed for one-step synthesis, it provides concise and direct routes for your target compounds, streamlining the synthesis process.
Accurate Predictions: Utilizing the extensive PISTACHIO, BKMS_METABOLIC, PISTACHIO_RINGBREAKER, REAXYS, REAXYS_BIOCATALYSIS database, our tool offers high-accuracy predictions, reflecting the latest in chemical research and data.
Strategy Settings
Precursor scoring | Relevance Heuristic |
---|---|
Min. plausibility | 0.01 |
Model | Template_relevance |
Template Set | Pistachio/Bkms_metabolic/Pistachio_ringbreaker/Reaxys/Reaxys_biocatalysis |
Top-N result to add to graph | 6 |
Feasible Synthetic Routes
Q1: What is the primary molecular target of geldanamycin?
A1: this compound specifically binds to and inhibits heat shock protein 90 (Hsp90) [, , , , , , , , ].
Q2: How does this compound interact with Hsp90?
A2: this compound binds to an atypical nucleotide-binding pocket in Hsp90, a site that shares homology with the adenosine triphosphate (ATP)-binding domain of bacterial DNA gyrase B []. This binding inhibits the essential ATPase activity of Hsp90 [].
Q3: What are the consequences of this compound binding to Hsp90?
A3: this compound binding disrupts Hsp90's chaperone function, leading to the destabilization, inactivation, and subsequent degradation of Hsp90 client proteins [, , ].
Q4: What are some examples of Hsp90 client proteins affected by this compound?
A4: this compound affects numerous signaling proteins crucial for cell growth, survival, and oncogenesis. Some examples include protein kinases like p185c-erbB-2, Src, Raf-1, Akt, and steroid hormone receptors [, , , , , , , ].
Q5: What are the downstream effects of this compound on cell signaling and survival?
A5: By promoting the degradation of Hsp90 client proteins, this compound disrupts various signaling pathways, leading to cell cycle arrest, apoptosis (programmed cell death), and inhibition of tumor cell proliferation [, , , , , ].
Q6: Does this compound affect the unfolded protein response (UPR)?
A6: Yes, this compound has been shown to influence the UPR. It can destabilize UPR signaling components like IRE1α and PERK by disrupting their interaction with HSP90, ultimately impacting the cellular response to ER stress [].
Q7: What is the molecular formula and weight of this compound?
A7: The molecular formula of this compound is C29H40N2O9, and its molecular weight is 560.64 g/mol.
Q8: What are the key structural features of this compound?
A8: this compound is a benzoquinone ansamycin, characterized by a benzoquinone ring system linked to a macrocyclic ansa bridge [, , , ].
Q9: Does this compound possess any inherent catalytic properties?
A9: While not a catalyst itself, this compound influences cellular processes by inhibiting the catalytic activity of Hsp90, thereby indirectly affecting the activities of various kinases and signaling proteins [].
Q10: Have computational methods been used to study this compound and its derivatives?
A10: Yes, computational methods like molecular docking and molecular dynamics simulations have been employed to understand the interactions of this compound and its derivatives with Hsp90, aiding in the design of novel inhibitors with improved properties [, ].
Q11: What are the key findings from computational studies on this compound binding to Hsp90?
A11: Molecular modeling studies have revealed that this compound binds to a specific pocket in the N-terminal domain of Hsp90, mimicking the binding mode of ATP []. This information is valuable for designing derivatives with enhanced binding affinity and specificity.
Q12: How does modifying the structure of this compound affect its activity?
A12: Modifications to the this compound scaffold have been explored to improve its pharmacological properties. For instance, introducing a 17-allylamino group (17-AAG) reduces toxicity while maintaining Hsp90 inhibitory activity [, , ].
Q13: What structural features of this compound are essential for its biological activity?
A13: The benzoquinone ring and the ansa bridge are crucial for this compound's interaction with Hsp90 []. Modifications to these regions can significantly impact its binding affinity and subsequent biological activity.
Q14: What are some examples of this compound derivatives, and how do their activities compare to the parent compound?
A14: Several this compound derivatives, such as 17-AAG (17-allylamino-17-demethoxythis compound), 17-DMAG (17-demethoxy-17-[[2-(dimethylamino)ethyl]amino]-geldanamycin), and 19-o-glycyl this compound, have been synthesized [, , , ]. These derivatives often exhibit altered pharmacological properties, including improved solubility, stability, and toxicity profiles compared to this compound.
Q15: What challenges are associated with the formulation of this compound?
A15: this compound exhibits limited water solubility, potentially hindering its bioavailability and clinical applications [].
Q16: How has the stability of this compound been addressed in derivative design?
A16: Derivatives like 17-DMAG have improved water solubility compared to this compound, potentially enhancing their bioavailability and suitability for intravenous administration [].
Q17: What is the role of chemical modifications in improving the stability of this compound?
A17: Chemical modifications can influence the stability of this compound under various conditions. For instance, certain derivatives exhibit increased photostability, making them more suitable for storage and handling [].
Q18: What is known about the absorption, distribution, metabolism, and excretion (ADME) of this compound?
A18: Although the provided abstracts do not detail specific ADME parameters, they highlight the exploration of derivatives like 17-DMAG with improved water solubility, which is expected to enhance absorption and potentially alter distribution profiles compared to this compound [].
Q19: How does the route of administration affect this compound's pharmacokinetic profile?
A19: The route of administration can significantly impact the pharmacokinetic properties of this compound and its analogs. While intravenous administration of soluble derivatives like 17-DMAG might provide favorable systemic exposure, alternative routes like oral administration may require specific formulations to overcome solubility challenges and achieve adequate bioavailability.
Q20: What types of in vitro assays are used to evaluate this compound's activity?
A20: Researchers use various in vitro assays, including cell proliferation assays (e.g., MTS assay), clonogenic assays, apoptosis assays (e.g., Annexin V staining), and invasion assays to assess the effects of this compound on cancer cell growth, survival, and metastatic potential [, , ].
Q21: What is the effect of this compound on tumor cells in vitro?
A21: this compound exhibits potent antiproliferative effects against various cancer cell lines in vitro, inducing cell cycle arrest and apoptosis, suggesting its potential as an anticancer agent [, , ].
Q22: What in vivo models have been used to study this compound's anticancer activity?
A22: this compound's anticancer properties have been investigated in vivo using xenograft mouse models, where human tumor cells are implanted into mice. These studies have demonstrated the compound's ability to inhibit tumor growth and metastasis [, ].
Q23: Has this compound been evaluated in clinical trials?
A23: While this compound itself faced challenges in clinical development due to solubility and toxicity issues, its derivative, 17-AAG, has undergone phase I clinical trials showing promising results [].
Q24: What were the key observations from 17-AAG clinical trials?
A24: Phase I clinical trials of 17-AAG demonstrated its potential in treating various cancers, including solid tumors and lymphoma []. These trials aimed to determine the maximum tolerated dose and evaluate safety and efficacy.
Q25: What are the known toxicities associated with this compound?
A25: this compound exhibits hepatotoxicity, limiting its clinical use [].
Q26: How does the toxicity of this compound derivatives compare to the parent compound?
A26: Derivatives like 17-AAG have been developed to address the toxicity concerns of this compound, displaying a more favorable safety profile [, ].
Q27: What strategies are being explored to mitigate the toxicity of this compound and its derivatives?
A27: Researchers are actively investigating ways to minimize the toxicity of this compound analogs. One approach involves developing derivatives with improved target specificity, aiming to reduce off-target effects and enhance their therapeutic window.
Q28: What is the impact of this compound's limited water solubility on its bioavailability?
A28: this compound's poor water solubility presents a significant challenge for its clinical use, potentially hindering its absorption and distribution in the body, ultimately affecting its bioavailability and therapeutic efficacy.
Q29: How does the solubility of this compound derivatives compare to the parent compound?
A29: Several derivatives, such as 17-DMAG, exhibit improved water solubility compared to this compound. This enhanced solubility is a desirable property for drug development, potentially leading to better absorption, distribution, and overall bioavailability.
Q30: Are there any alternative compounds with similar biological activity to this compound?
A30: Yes, radicicol, another natural product, also binds to the ATP-binding site of Hsp90 and disrupts its function, exhibiting similar effects to this compound [].
Q31: What are the advantages and disadvantages of using alternative Hsp90 inhibitors compared to this compound?
A31: While both this compound and radicicol effectively inhibit Hsp90, they differ in their pharmacokinetic properties and toxicity profiles. Radicicol, for instance, exhibits greater instability in vivo, while this compound raises concerns regarding hepatotoxicity. The choice between these inhibitors depends on the specific application and desired therapeutic window.
Q32: When was this compound first discovered, and what were its initial applications?
A32: this compound was initially isolated as a natural product with antifungal activity [].
Q33: How has the understanding of this compound's mechanism of action evolved over time?
A33: Early research primarily focused on this compound's antifungal properties. Subsequent investigations unveiled its ability to inhibit the proliferation of cancer cells transformed by oncogene kinases, leading to the discovery of Hsp90 as its primary molecular target []. This finding marked a significant milestone, paving the way for exploring this compound and its analogs as potential anticancer agents.
体外研究产品的免责声明和信息
请注意,BenchChem 上展示的所有文章和产品信息仅供信息参考。 BenchChem 上可购买的产品专为体外研究设计,这些研究在生物体外进行。体外研究,源自拉丁语 "in glass",涉及在受控实验室环境中使用细胞或组织进行的实验。重要的是要注意,这些产品没有被归类为药物或药品,他们没有得到 FDA 的批准,用于预防、治疗或治愈任何医疗状况、疾病或疾病。我们必须强调,将这些产品以任何形式引入人类或动物的身体都是法律严格禁止的。遵守这些指南对确保研究和实验的法律和道德标准的符合性至关重要。