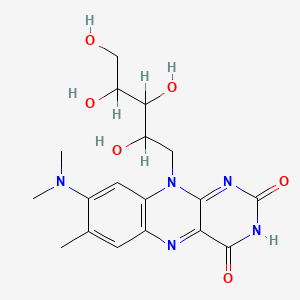
玫瑰黄素
描述
Roseoflavin is a naturally occurring riboflavin (vitamin B2) analogue with significant antibiotic properties. It is produced by the bacteria Streptomyces davaonensis and Streptomyces cinnabarinus. Roseoflavin is unique as it is the only known natural riboflavin analogue with antibiotic activity, making it a promising candidate for various scientific and medical applications .
科学研究应用
Roseoflavin has a wide range of scientific research applications:
Chemistry: Roseoflavin is used as a model compound to study the properties and reactions of riboflavin analogues.
Biology: It serves as a tool to investigate the metabolic pathways involving riboflavin and its analogues.
Medicine: Roseoflavin has shown potential as an antimicrobial agent against various bacteria, including Staphylococcus aureus, Enterococcus faecalis, and Streptococcus pyogenes.
作用机制
Target of Action
Roseoflavin, a natural riboflavin (vitamin B2) analogue, primarily targets the metabolism of riboflavin and the utilization of riboflavin metabolites . The key enzymes responsible for roseoflavin biosynthesis have been identified . These include the enzyme encoded by the gene rosB
, which converts flavin mononucleotide (FMN) to 8-demethyl-8-aminoriboflavin-5’-phosphate .
Mode of Action
Roseoflavin interacts with its targets by inhibiting the production of flavin mononucleotide (FMN) and flavin adenine dinucleotide (FAD), essential cofactors in various biochemical reactions . It binds to FMN riboswitches, thereby regulating gene expression .
Biochemical Pathways
The biochemical pathway of roseoflavin biosynthesis starts from flavin mononucleotide (FMN) and proceeds through an immediate precursor, aminoriboflavin . The enzyme encoded by the gene rosB
converts FMN to 8-demethyl-8-aminoriboflavin-5’-phosphate (aminoriboflavin-5’-phosphate, AFP). AFP is then dephosphorylated to aminoriboflavin (AF) by a specific phosphatase, the product of the gene rosC
. Finally, AF is dimethylated to roseoflavin using an enzyme encoded by rosA
.
Pharmacokinetics
It is known that roseoflavin is naturally produced by certain bacteria
Result of Action
The molecular and cellular effects of roseoflavin’s action include the inhibition of growth in various bacteria and protozoal parasites . For instance, roseoflavin has been found to inhibit the growth of Staphylococcus aureus, Enterococcus faecalis, Streptococcus pyogenes, Listeria monocytogenes, and parasites like Trypanosoma cruzi, Trypanosoma brucei, and Leishmania mexicana .
Action Environment
It is known that roseoflavin is used in the food industry to select roseoflavin-resistant strains , suggesting that its action can be influenced by the presence of other microbial species
生化分析
Biochemical Properties
Roseoflavin plays a significant role in biochemical reactions due to its antibiotic properties. It interacts with various enzymes, proteins, and other biomolecules. The biosynthesis of roseoflavin involves several key enzymes, including the enzyme encoded by the gene rosB, which converts flavin mononucleotide to 8-demethyl-8-aminoriboflavin-5’-phosphate . This intermediate is then dephosphorylated to aminoriboflavin by a specific phosphatase encoded by the gene rosC . Finally, aminoriboflavin is dimethylated to roseoflavin using an enzyme encoded by the gene rosA . These interactions highlight the complex biochemical pathways involved in the production of roseoflavin.
Cellular Effects
Roseoflavin exerts various effects on different types of cells and cellular processes. It has been shown to inhibit the growth of several bacterial strains, including Staphylococcus aureus and Listeria monocytogenes . Roseoflavin influences cell function by interfering with cell signaling pathways, gene expression, and cellular metabolism. For instance, it can disrupt the normal functioning of riboflavin-dependent enzymes, leading to altered metabolic processes and impaired bacterial growth .
Molecular Mechanism
The molecular mechanism of action of roseoflavin involves its interaction with riboflavin-dependent enzymes. Roseoflavin acts as an antimetabolite by mimicking riboflavin and competitively inhibiting riboflavin-dependent enzymes . This inhibition disrupts the normal catalytic activities of these enzymes, leading to a cascade of effects on cellular metabolism and gene expression. Additionally, roseoflavin can bind to riboflavin transporters, further inhibiting the uptake of riboflavin by bacterial cells .
Temporal Effects in Laboratory Settings
In laboratory settings, the effects of roseoflavin have been observed to change over time. Roseoflavin is relatively stable under standard laboratory conditions, but it can degrade over extended periods . Long-term studies have shown that roseoflavin can have sustained inhibitory effects on bacterial growth, although the extent of inhibition may decrease over time due to degradation . These temporal effects highlight the importance of considering the stability and degradation of roseoflavin in experimental designs.
Dosage Effects in Animal Models
The effects of roseoflavin vary with different dosages in animal models. At lower doses, roseoflavin has been shown to effectively inhibit bacterial growth without causing significant toxic effects . At higher doses, roseoflavin can exhibit toxic or adverse effects, including potential damage to host tissues . These dosage-dependent effects underscore the need for careful optimization of roseoflavin dosages in therapeutic applications.
Metabolic Pathways
Roseoflavin is involved in several metabolic pathways, primarily related to its role as an antimetabolite. It interacts with enzymes involved in riboflavin metabolism, such as riboflavin kinase and flavokinase . By inhibiting these enzymes, roseoflavin disrupts the normal metabolic flux of riboflavin and its derivatives, leading to altered levels of metabolites and impaired cellular functions . These interactions highlight the intricate metabolic pathways influenced by roseoflavin.
Transport and Distribution
Within cells and tissues, roseoflavin is transported and distributed through specific transporters and binding proteins. It can interact with riboflavin transporters, affecting its localization and accumulation within cells . The distribution of roseoflavin is influenced by its binding affinity to these transporters, which can impact its overall efficacy and bioavailability . Understanding the transport and distribution mechanisms of roseoflavin is crucial for optimizing its therapeutic potential.
Subcellular Localization
Roseoflavin exhibits specific subcellular localization patterns that influence its activity and function. It can be targeted to specific compartments or organelles through post-translational modifications and targeting signals . These localization patterns are essential for understanding the precise mechanisms by which roseoflavin exerts its effects within cells. By studying the subcellular localization of roseoflavin, researchers can gain insights into its mode of action and potential therapeutic applications.
准备方法
Synthetic Routes and Reaction Conditions: Roseoflavin is primarily produced through chemical synthesis. The synthesis begins with the nitration of N,N-dimethyl-o-toluidine to form 2-dimethylamino-4-nitrotoluene. This compound is then reduced using hydrazine in the presence of Raney nickel to yield 2-dimethylamino-4-aminotoluene. The next step involves the condensation of this compound with D-ribose, followed by pressure hydrogenation in the presence of Raney nickel to produce 2-dimethylamino-4-o-ribitylaminotoluene. This intermediate is then condensed with violuric acid to form roseoflavin. The crude product is acetylated to form roseoflavin-tetraacetate, which is then recrystallized from methanol. Finally, the purified acetate is hydrolyzed with sodium hydroxide to yield roseoflavin .
Industrial Production Methods: Industrial production of roseoflavin involves the use of genetically engineered microorganisms. For instance, the genes responsible for roseoflavin biosynthesis have been successfully expressed in Corynebacterium glutamicum and Streptomyces davaonensis, leading to enhanced production yields. These methods provide a more economical and scalable approach to producing roseoflavin .
化学反应分析
Types of Reactions: Roseoflavin undergoes various chemical reactions, including oxidation, reduction, and substitution reactions.
Common Reagents and Conditions:
Oxidation: Roseoflavin can be oxidized using common oxidizing agents such as hydrogen peroxide or potassium permanganate.
Reduction: Reduction of roseoflavin can be achieved using reducing agents like sodium borohydride or lithium aluminum hydride.
Substitution: Substitution reactions involving roseoflavin typically require nucleophilic reagents under basic conditions.
Major Products: The major products formed from these reactions depend on the specific reagents and conditions used. For example, oxidation of roseoflavin can yield various oxidized derivatives, while reduction can produce reduced forms of the compound .
相似化合物的比较
Riboflavin: The parent compound of roseoflavin, riboflavin, is a vital nutrient involved in various cellular processes.
8-Aminoriboflavin: Another riboflavin analogue with similar properties to roseoflavin, but with different biological activities.
Uniqueness of Roseoflavin: Roseoflavin is unique due to its antibiotic properties, which are not observed in other riboflavin analogues. Its ability to bind to flavin mononucleotide riboswitches and inhibit essential bacterial processes makes it a valuable compound for antimicrobial research and potential therapeutic applications .
属性
IUPAC Name |
8-(dimethylamino)-7-methyl-10-(2,3,4,5-tetrahydroxypentyl)benzo[g]pteridine-2,4-dione | |
---|---|---|
Source | PubChem | |
URL | https://pubchem.ncbi.nlm.nih.gov | |
Description | Data deposited in or computed by PubChem | |
InChI |
InChI=1S/C18H23N5O6/c1-8-4-9-11(5-10(8)22(2)3)23(6-12(25)15(27)13(26)7-24)16-14(19-9)17(28)21-18(29)20-16/h4-5,12-13,15,24-27H,6-7H2,1-3H3,(H,21,28,29) | |
Source | PubChem | |
URL | https://pubchem.ncbi.nlm.nih.gov | |
Description | Data deposited in or computed by PubChem | |
InChI Key |
IGQLDUYTWDABFK-UHFFFAOYSA-N | |
Source | PubChem | |
URL | https://pubchem.ncbi.nlm.nih.gov | |
Description | Data deposited in or computed by PubChem | |
Canonical SMILES |
CC1=CC2=C(C=C1N(C)C)N(C3=NC(=O)NC(=O)C3=N2)CC(C(C(CO)O)O)O | |
Source | PubChem | |
URL | https://pubchem.ncbi.nlm.nih.gov | |
Description | Data deposited in or computed by PubChem | |
Molecular Formula |
C18H23N5O6 | |
Source | PubChem | |
URL | https://pubchem.ncbi.nlm.nih.gov | |
Description | Data deposited in or computed by PubChem | |
DSSTOX Substance ID |
DTXSID60965368 | |
Record name | 1-Deoxy-1-[8-(dimethylamino)-4-hydroxy-7-methyl-2-oxobenzo[g]pteridin-10(2H)-yl]pentitol | |
Source | EPA DSSTox | |
URL | https://comptox.epa.gov/dashboard/DTXSID60965368 | |
Description | DSSTox provides a high quality public chemistry resource for supporting improved predictive toxicology. | |
Molecular Weight |
405.4 g/mol | |
Source | PubChem | |
URL | https://pubchem.ncbi.nlm.nih.gov | |
Description | Data deposited in or computed by PubChem | |
CAS No. |
51093-55-1 | |
Record name | Roseoflavin | |
Source | ChemIDplus | |
URL | https://pubchem.ncbi.nlm.nih.gov/substance/?source=chemidplus&sourceid=0051093551 | |
Description | ChemIDplus is a free, web search system that provides access to the structure and nomenclature authority files used for the identification of chemical substances cited in National Library of Medicine (NLM) databases, including the TOXNET system. | |
Record name | 1-Deoxy-1-[8-(dimethylamino)-4-hydroxy-7-methyl-2-oxobenzo[g]pteridin-10(2H)-yl]pentitol | |
Source | EPA DSSTox | |
URL | https://comptox.epa.gov/dashboard/DTXSID60965368 | |
Description | DSSTox provides a high quality public chemistry resource for supporting improved predictive toxicology. | |
Retrosynthesis Analysis
AI-Powered Synthesis Planning: Our tool employs the Template_relevance Pistachio, Template_relevance Bkms_metabolic, Template_relevance Pistachio_ringbreaker, Template_relevance Reaxys, Template_relevance Reaxys_biocatalysis model, leveraging a vast database of chemical reactions to predict feasible synthetic routes.
One-Step Synthesis Focus: Specifically designed for one-step synthesis, it provides concise and direct routes for your target compounds, streamlining the synthesis process.
Accurate Predictions: Utilizing the extensive PISTACHIO, BKMS_METABOLIC, PISTACHIO_RINGBREAKER, REAXYS, REAXYS_BIOCATALYSIS database, our tool offers high-accuracy predictions, reflecting the latest in chemical research and data.
Strategy Settings
Precursor scoring | Relevance Heuristic |
---|---|
Min. plausibility | 0.01 |
Model | Template_relevance |
Template Set | Pistachio/Bkms_metabolic/Pistachio_ringbreaker/Reaxys/Reaxys_biocatalysis |
Top-N result to add to graph | 6 |
Feasible Synthetic Routes
体外研究产品的免责声明和信息
请注意,BenchChem 上展示的所有文章和产品信息仅供信息参考。 BenchChem 上可购买的产品专为体外研究设计,这些研究在生物体外进行。体外研究,源自拉丁语 "in glass",涉及在受控实验室环境中使用细胞或组织进行的实验。重要的是要注意,这些产品没有被归类为药物或药品,他们没有得到 FDA 的批准,用于预防、治疗或治愈任何医疗状况、疾病或疾病。我们必须强调,将这些产品以任何形式引入人类或动物的身体都是法律严格禁止的。遵守这些指南对确保研究和实验的法律和道德标准的符合性至关重要。