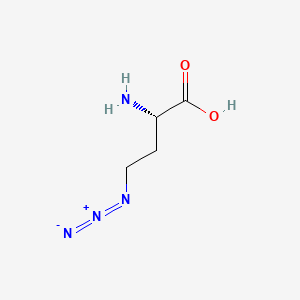
(S)-2-Amino-4-azidobutanoic acid
描述
L-Azidohomoalanine HCl salt is an amino acid analog that provides a fast, sensitive, non-toxic and non-radioactive alternative to the traditional radioactive 35S-methionine for the detection of nascent protein. L-azidohomoalaine contains a small modification and its azido moiety can be fed to cultured cells and incorporated into proteins during active protein synthesis.
作用机制
Target of Action
L-Azidohomoalanine (AHA) is an amino acid analog of methionine . It is primarily targeted towards proteins during active protein synthesis . The azido moiety in AHA can be incorporated into proteins, replacing methionine .
Mode of Action
The mode of action of AHA involves its incorporation into proteins during active protein synthesis . This is achieved through the chemoselective ligation or “click” reaction between an azide and an alkyne or cyclooctyne . The azido-modified protein can then be detected with either a fluorescent alkyne or a biotin alkyne .
Biochemical Pathways
The biochemical pathway of AHA involves its intracellular biosynthesis from O-acetyl-L-homoserine and NaN3 . It is then incorporated into recombinant target proteins by AUG codon reassignment in a methionine-auxotroph E. coli strain .
Result of Action
The result of AHA’s action is the creation of azido-modified proteins. These proteins can be detected with either fluorescent alkyne or biotin alkyne . The detection sensitivity with these reagents in 1-D gels and Western blots is in the low femtomole range and is compatible with downstream LC-MS/MS and MALDI-MS analysis .
Action Environment
The action environment of AHA is primarily within cultured cells where it is incorporated into proteins during active protein synthesis . The efficiency of this process can be influenced by various factors, including the concentration of AHA and the presence of other amino acids.
生化分析
Biochemical Properties
L-Azidohomoalanine can substitute for L-methionine as a substrate for Escherichia coli methionyl tRNA synthetase and is globally incorporated instead of L-methionine in the E. coli outer membrane protein OmpC when expressed in auxotrophic E. coli . This property allows L-Azidohomoalanine to interact with a wide range of enzymes, proteins, and other biomolecules, and the nature of these interactions is largely determined by the specific biochemical context.
Cellular Effects
The incorporation of L-Azidohomoalanine into proteins can have various effects on cellular processes. For instance, it has been used to study protein dynamics in development and disease . It can influence cell function by altering the properties of the proteins into which it is incorporated. This can impact cell signaling pathways, gene expression, and cellular metabolism .
Molecular Mechanism
L-Azidohomoalanine exerts its effects at the molecular level through its incorporation into proteins. It can participate in bioorthogonal reactions such as copper-mediated Huisgen-[3 + 2]-cycloadditions .
Temporal Effects in Laboratory Settings
The effects of L-Azidohomoalanine can change over time in laboratory settings. For example, it has been used in pulse-chase experiments to study the dynamics of protein synthesis and degradation . Information on the product’s stability, degradation, and long-term effects on cellular function can be obtained from such studies .
Dosage Effects in Animal Models
The effects of L-Azidohomoalanine can vary with different dosages in animal models. For instance, it has been demonstrated that labeling the murine proteome is feasible via injection of L-Azidohomoalanine without the need for methionine depletion . The study also showed that L-Azidohomoalanine administration does not significantly perturb cellular functions as reflected by an unchanged plasma metabolome compared to non-injected controls .
Metabolic Pathways
L-Azidohomoalanine is involved in the metabolic pathway of protein synthesis, where it can be incorporated into proteins in place of methionine . It interacts with the enzyme methionyl tRNA synthetase, which catalyzes the attachment of methionine to its cognate tRNA .
Transport and Distribution
L-Azidohomoalanine can be transported and distributed within cells and tissues. For instance, it has been shown that L-Azidohomoalanine can be injected into mice and distributed in various tissues, where it is incorporated into proteins .
Subcellular Localization
The subcellular localization of L-Azidohomoalanine is determined by the proteins into which it is incorporated. Since it can be incorporated into any protein that normally contains methionine, it can potentially be found in any subcellular compartment or organelle where such proteins are located .
生物活性
(S)-2-Amino-4-azidobutanoic acid, also known as S-A4A, is an amino acid derivative characterized by the presence of an azido group (-N₃) attached to the butanoic backbone. This unique structure provides the compound with distinctive biological properties and applications, particularly in the fields of protein engineering and drug development.
- Molecular Formula : C₄H₈N₄O₂
- Molecular Weight : 144.13 g/mol
- Structure : The compound features an azido group which is crucial for its reactivity and biological activity.
While this compound does not have a well-defined mechanism of action in biological systems, its primary role is as a tool in protein engineering. The azide group allows for:
- Site-Specific Mutations : When incorporated into proteins during cell culture experiments, S-A4A can introduce mutations at specific sites, enabling researchers to study the functional consequences of these mutations.
- Protein Labeling : The azido group can be utilized in click chemistry for protein labeling, facilitating visualization and tracking of protein interactions.
- Bioorthogonal Reactions : S-A4A participates in bioorthogonal reactions that occur without interfering with native cellular processes, allowing for selective conjugation with other biomolecules under physiological conditions.
1. Mutagenicity and Genetic Studies
Research indicates that this compound exhibits mutagenic properties, which may have implications for genetic studies and drug development. Its ability to modify nucleic acids could be leveraged in targeted therapies or investigations into gene expression mechanisms.
2. Interaction Studies
Interaction studies involving S-A4A focus on its capacity to bind various biological targets. Preliminary research suggests that it can influence gene expression and stability by interacting with nucleic acids. Ongoing investigations aim to clarify its interactions with proteins and other biomolecules.
Comparative Analysis
The following table compares this compound with structurally similar compounds:
Compound | Structural Features | Unique Properties |
---|---|---|
This compound | Azido group on butanoic backbone | Mutagenicity, potential drug development |
L-Homoserine | No azido group | Precursor for various amino acids |
4-Azido-L-homoalanine | Azido group on homoalanine backbone | Similar mutagenicity but different applications |
L-Azidoalanine | Azido group on alanine | Different biological activity than S-A4A |
Case Study 1: Protein Engineering
In a study utilizing superfolder-GFP (sfGFP) as a model protein, researchers demonstrated the incorporation efficiency of non-canonical amino acids (ncAAs), including S-A4A. High concentrations of ncAAs were employed to ensure detection of even low incorporation activity, highlighting the utility of S-A4A in probing protein function through site-specific modifications .
Case Study 2: Drug Development
The unique structure of this compound has been explored for developing peptide-based drugs targeting specific biological pathways. Its incorporation into peptides facilitates the design of therapeutics that can engage with cellular mechanisms more effectively than traditional compounds .
科学研究应用
Peptide Synthesis
Role in Solid-Phase Peptide Synthesis
(S)-2-Amino-4-azidobutanoic acid serves as a crucial building block in the synthesis of peptides. Its azido functionality allows for the incorporation of this amino acid into peptide chains during solid-phase synthesis. This incorporation can be utilized to create peptides with specific properties or functionalities that are beneficial for therapeutic applications .
Case Study: Synthesis of Therapeutic Peptides
Recent studies have demonstrated the utility of this compound in synthesizing peptides that target specific biological pathways. For instance, researchers have successfully synthesized peptide analogs that exhibit enhanced binding affinities to their targets due to the azido group’s ability to facilitate further modifications .
Drug Development
Facilitating New Therapeutic Agents
The unique structure of this compound enables researchers to explore new therapeutic agents. The azido group can be modified through various chemical reactions, leading to the development of peptide-based drugs that can effectively target diseases .
Case Study: Targeted Drug Delivery Systems
In drug development, this compound has been employed in creating targeted drug delivery systems. By conjugating this compound with therapeutic agents, researchers have been able to enhance the specificity and efficacy of drugs, reducing side effects and improving patient outcomes .
Bioconjugation and Click Chemistry
Bioorthogonal Reactions
The azide functionality of this compound is particularly valuable in bioorthogonal chemistry. It allows for selective conjugation with biomolecules containing complementary functional groups without interfering with native cellular processes. This property makes it an ideal candidate for labeling proteins and studying protein interactions .
Case Study: Protein Labeling Techniques
Researchers have utilized this compound in click chemistry approaches for protein labeling. By attaching biocompatible molecules to the azide group, they have successfully visualized protein interactions within live cells, providing insights into cellular mechanisms and protein dynamics .
Protein Engineering
Modifying Protein Functions
In protein engineering, this compound can be incorporated into proteins to introduce site-specific mutations. This capability allows scientists to study the functional consequences of these mutations on protein behavior and stability, paving the way for the development of more effective biopharmaceuticals .
Data Table: Summary of Applications
Application Area | Description | Key Benefits |
---|---|---|
Peptide Synthesis | Building block for solid-phase peptide synthesis | Enables targeted modifications |
Drug Development | Facilitates creation of peptide-based therapeutic agents | Improves specificity and efficacy |
Bioconjugation | Utilized in click chemistry for selective biomolecule conjugation | Non-invasive tracking of protein interactions |
Protein Engineering | Introduces mutations for functional studies | Enhances understanding of protein dynamics |
Genetic Studies
Mutagenesis Studies
this compound exhibits mutagenic properties, which can be leveraged in genetic studies. Its ability to modify nucleic acids may lead to advancements in targeted therapies and gene expression research .
属性
IUPAC Name |
(2S)-2-amino-4-azidobutanoic acid | |
---|---|---|
Source | PubChem | |
URL | https://pubchem.ncbi.nlm.nih.gov | |
Description | Data deposited in or computed by PubChem | |
InChI |
InChI=1S/C4H8N4O2/c5-3(4(9)10)1-2-7-8-6/h3H,1-2,5H2,(H,9,10)/t3-/m0/s1 | |
Source | PubChem | |
URL | https://pubchem.ncbi.nlm.nih.gov | |
Description | Data deposited in or computed by PubChem | |
InChI Key |
NNWQLZWAZSJGLY-VKHMYHEASA-N | |
Source | PubChem | |
URL | https://pubchem.ncbi.nlm.nih.gov | |
Description | Data deposited in or computed by PubChem | |
Canonical SMILES |
C(CN=[N+]=[N-])C(C(=O)O)N | |
Source | PubChem | |
URL | https://pubchem.ncbi.nlm.nih.gov | |
Description | Data deposited in or computed by PubChem | |
Isomeric SMILES |
C(CN=[N+]=[N-])[C@@H](C(=O)O)N | |
Source | PubChem | |
URL | https://pubchem.ncbi.nlm.nih.gov | |
Description | Data deposited in or computed by PubChem | |
Molecular Formula |
C4H8N4O2 | |
Source | PubChem | |
URL | https://pubchem.ncbi.nlm.nih.gov | |
Description | Data deposited in or computed by PubChem | |
DSSTOX Substance ID |
DTXSID50152664 | |
Record name | (S)-2-Amino-4-azidobutanoic acid | |
Source | EPA DSSTox | |
URL | https://comptox.epa.gov/dashboard/DTXSID50152664 | |
Description | DSSTox provides a high quality public chemistry resource for supporting improved predictive toxicology. | |
Molecular Weight |
144.13 g/mol | |
Source | PubChem | |
URL | https://pubchem.ncbi.nlm.nih.gov | |
Description | Data deposited in or computed by PubChem | |
CAS No. |
120042-14-0 | |
Record name | (S)-2-Amino-4-azidobutanoic acid | |
Source | ChemIDplus | |
URL | https://pubchem.ncbi.nlm.nih.gov/substance/?source=chemidplus&sourceid=0120042140 | |
Description | ChemIDplus is a free, web search system that provides access to the structure and nomenclature authority files used for the identification of chemical substances cited in National Library of Medicine (NLM) databases, including the TOXNET system. | |
Record name | (S)-2-Amino-4-azidobutanoic acid | |
Source | EPA DSSTox | |
URL | https://comptox.epa.gov/dashboard/DTXSID50152664 | |
Description | DSSTox provides a high quality public chemistry resource for supporting improved predictive toxicology. | |
Retrosynthesis Analysis
AI-Powered Synthesis Planning: Our tool employs the Template_relevance Pistachio, Template_relevance Bkms_metabolic, Template_relevance Pistachio_ringbreaker, Template_relevance Reaxys, Template_relevance Reaxys_biocatalysis model, leveraging a vast database of chemical reactions to predict feasible synthetic routes.
One-Step Synthesis Focus: Specifically designed for one-step synthesis, it provides concise and direct routes for your target compounds, streamlining the synthesis process.
Accurate Predictions: Utilizing the extensive PISTACHIO, BKMS_METABOLIC, PISTACHIO_RINGBREAKER, REAXYS, REAXYS_BIOCATALYSIS database, our tool offers high-accuracy predictions, reflecting the latest in chemical research and data.
Strategy Settings
Precursor scoring | Relevance Heuristic |
---|---|
Min. plausibility | 0.01 |
Model | Template_relevance |
Template Set | Pistachio/Bkms_metabolic/Pistachio_ringbreaker/Reaxys/Reaxys_biocatalysis |
Top-N result to add to graph | 6 |
Feasible Synthetic Routes
Q1: How does AHA get incorporated into proteins?
A1: AHA mimics the structure of the amino acid methionine and is recognized by the cellular machinery responsible for protein synthesis. [, , , ]. During translation, AHA is incorporated into nascent polypeptide chains in place of methionine [, , , , ].
Q2: How does AHA help study protein synthesis and degradation?
A2: AHA serves as a marker for newly synthesized proteins. By introducing AHA for a specific time (pulse), researchers can track the fate of these proteins, providing insights into protein synthesis rates, degradation pathways (like autophagy), and protein half-life [, , ].
Q3: What is the molecular formula and weight of AHA?
A3: The molecular formula of AHA is C4H8N4O2, and its molecular weight is 144.13 g/mol.
Q4: What spectroscopic data is available for AHA?
A4: Spectroscopic data, such as NMR and mass spectrometry, are crucial for confirming AHA incorporation into proteins and characterizing its structure [, , ]. For specific details, please refer to individual publications focusing on AHA synthesis and characterization [].
Q5: Is AHA compatible with live cells?
A5: Yes, AHA exhibits good biocompatibility and can be used to label newly synthesized proteins in a variety of living cells, including bacteria, yeast, and mammalian cells [, , , , ].
Q6: Does AHA have any inherent catalytic properties?
A6: No, AHA itself does not possess catalytic properties. It is primarily used as a tool for bioconjugation and protein labeling due to the reactivity of its azide group.
Q7: How is AHA utilized in bioorthogonal reactions?
A7: The azide group of AHA readily participates in click chemistry reactions, specifically the copper-catalyzed azide-alkyne cycloaddition (CuAAC) or the strain-promoted azide-alkyne cycloaddition (SPAAC) [, , , , ]. These reactions allow for the covalent attachment of various probes or tags to AHA-labeled proteins, enabling visualization, purification, or further analysis.
Q8: Are there computational studies on AHA incorporation?
A8: While computational studies specifically focusing on AHA incorporation are limited, molecular modeling techniques can be employed to understand its interaction with the ribosome and potential effects on protein structure.
Q9: How do structural modifications of AHA affect its activity?
A9: Structural modifications to AHA are primarily focused on altering the linker between the amino acid backbone and the azide group, influencing its reactivity in click reactions or its ability to be recognized by the translational machinery.
Q10: What are the formulation strategies for AHA?
A10: AHA is typically supplied as a solid and dissolved in appropriate solvents for experimental use. Its formulation can be optimized for specific applications, considering factors like solubility, stability, and compatibility with downstream analyses.
Q11: What are the safety concerns associated with AHA?
A11: While AHA is generally considered safe for laboratory use, standard safety precautions should be followed when handling any chemical. Always refer to the material safety data sheet (MSDS) provided by the supplier for specific information.
Q12: What are the limitations of AHA in in vivo studies?
A12: AHA's application in in vivo studies can be limited by its potential metabolism or clearance from the system. Moreover, the requirement for a click reaction to visualize labeled proteins can pose challenges for deep tissue imaging in live animals.
Q13: How is AHA quantified in biological samples?
A13: AHA itself is not directly quantified in biological samples. Its incorporation into proteins is assessed indirectly by detecting the probes or tags attached through click chemistry reactions.
Q14: Are there alternatives to AHA for studying protein synthesis?
A14: Yes, alternative methods for studying protein synthesis include stable isotope labeling by amino acids in cell culture (SILAC), radioisotope labeling with 35S-methionine, and puromycin labeling [, ]. Each method has its advantages and limitations, and the choice depends on the specific research question and experimental system.
体外研究产品的免责声明和信息
请注意,BenchChem 上展示的所有文章和产品信息仅供信息参考。 BenchChem 上可购买的产品专为体外研究设计,这些研究在生物体外进行。体外研究,源自拉丁语 "in glass",涉及在受控实验室环境中使用细胞或组织进行的实验。重要的是要注意,这些产品没有被归类为药物或药品,他们没有得到 FDA 的批准,用于预防、治疗或治愈任何医疗状况、疾病或疾病。我们必须强调,将这些产品以任何形式引入人类或动物的身体都是法律严格禁止的。遵守这些指南对确保研究和实验的法律和道德标准的符合性至关重要。