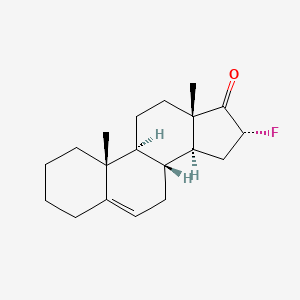
氟醋酸酯
描述
科学研究应用
Chemistry: Used as a model compound to study fluorinated steroids.
Biology: Investigated for its effects on enzyme activity and gene expression.
Medicine: Explored for its anti-inflammatory, anti-proliferative, and anti-diabetic properties.
作用机制
Target of Action
Fluasterone is a synthetically stable adrenocortical steroid fluorinated analogue of dehydroepiandrosterone (DHEA), a powerful anti-inflammatory molecule .
Mode of Action
Fluasterone interacts with its targets by inhibiting NF-kB activation and reducing oxidative stress . This interaction results in the suppression of inflammation, making it effective in preclinical models of chronic inflammatory diseases including psoriasis, asthma, rheumatoid arthritis, multiple sclerosis, and lupus erythematosus .
Biochemical Pathways
It is known that fluasterone has a potent uncompetitive inhibitory effect on glucose-6-phosphate dehydrogenase (g6pdh), which is stronger than that of dhea . This inhibition may play a role in its anti-inflammatory, antihyperplastic, chemopreventative, antihyperlipidemic, antidiabetic, and antiobesic activities .
Pharmacokinetics
It is known that due to extensive first-pass hepatic and/or gastrointestinal metabolism, very high doses of fluasterone are necessary for effectiveness .
Result of Action
The molecular and cellular effects of Fluasterone’s action include the suppression of inflammation and the reduction of oxidative stress . It has shown effectiveness in pre
生化分析
Biochemical Properties
Fluasterone is a potent uncompetitive inhibitor of glucose-6-phosphate dehydrogenase (G6PDH) . It retains the anti-inflammatory, antihyperplastic, chemopreventative, antihyperlipidemic, antidiabetic, and antiobesic, as well as certain immunomodulating activities of DHEA .
Cellular Effects
Fluasterone has been shown to inhibit the activity and expression of carcinogen-activating enzymes in MCF-7 cells . It also exhibits DHEA-like effects, including anti-inflammatory, anti-proliferative, and anti-diabetic properties but is consistently more potent than DHEA .
Molecular Mechanism
It is proposed that Fluasterone inhibits NF-kB activation and reduces oxidative stress . Unlike DHEA, Fluasterone has minimal or no androgenic or estrogenic activity .
Temporal Effects in Laboratory Settings
In animal models, Fluasterone exhibits DHEA-like effects, including anti-inflammatory, anti-proliferative, and anti-diabetic properties . Over time, these effects have been observed to be consistently more potent than DHEA .
Dosage Effects in Animal Models
In animal models, Fluasterone, when injected subcutaneously at 5 mg/kg, decreased plasma corticosterone levels, and this was correlated with lowering of fasting plasma glucose . As the dose of Fluasterone was increased, corticosterone and fasting plasma glucose levels both rebounded due to increased corticotropin .
Metabolic Pathways
It is known that Fluasterone is a potent uncompetitive inhibitor of G6PDH .
Transport and Distribution
Due to extensive first-pass hepatic and/or gastrointestinal metabolism, very high doses of DHEA and Fluasterone are necessary for effectiveness . This suggests that Fluasterone is extensively metabolized in the liver and gastrointestinal tract.
准备方法
Fluasterone is synthesized by modifying DHEA. The synthetic route involves the removal of the C3β hydroxyl group and the substitution of a hydrogen atom with a fluorine atom at the C16α position . Specific reaction conditions and industrial production methods are not widely documented, but the process generally involves standard organic synthesis techniques, including fluorination and dehydroxylation reactions .
化学反应分析
Fluasterone undergoes various chemical reactions, including:
Oxidation: Fluasterone can be oxidized to form different derivatives.
Reduction: Reduction reactions can modify the ketone group at the C17 position.
Substitution: The fluorine atom at the C16α position can be involved in substitution reactions.
Common reagents used in these reactions include oxidizing agents like potassium permanganate and reducing agents like sodium borohydride. The major products formed depend on the specific reaction conditions and reagents used .
相似化合物的比较
Fluasterone is compared with other DHEA analogues, such as:
Dehydroepiandrosterone (DHEA): Fluasterone retains the beneficial properties of DHEA but with reduced androgenic and estrogenic activities.
16α-Fluoro-5-androsten-17-one: Another fluorinated analogue with similar properties but different metabolic pathways.
Fluasterone’s uniqueness lies in its potent inhibition of G6PDH and its minimal androgenic and estrogenic activities, making it a safer alternative for therapeutic applications .
属性
IUPAC Name |
(8R,9S,10R,13S,14S,16R)-16-fluoro-10,13-dimethyl-1,2,3,4,7,8,9,11,12,14,15,16-dodecahydrocyclopenta[a]phenanthren-17-one | |
---|---|---|
Source | PubChem | |
URL | https://pubchem.ncbi.nlm.nih.gov | |
Description | Data deposited in or computed by PubChem | |
InChI |
InChI=1S/C19H27FO/c1-18-9-4-3-5-12(18)6-7-13-14(18)8-10-19(2)15(13)11-16(20)17(19)21/h6,13-16H,3-5,7-11H2,1-2H3/t13-,14+,15+,16-,18+,19+/m1/s1 | |
Source | PubChem | |
URL | https://pubchem.ncbi.nlm.nih.gov | |
Description | Data deposited in or computed by PubChem | |
InChI Key |
VHZXNQKVFDBFIK-NBBHSKLNSA-N | |
Source | PubChem | |
URL | https://pubchem.ncbi.nlm.nih.gov | |
Description | Data deposited in or computed by PubChem | |
Canonical SMILES |
CC12CCCCC1=CCC3C2CCC4(C3CC(C4=O)F)C | |
Source | PubChem | |
URL | https://pubchem.ncbi.nlm.nih.gov | |
Description | Data deposited in or computed by PubChem | |
Isomeric SMILES |
C[C@]12CCCCC1=CC[C@@H]3[C@@H]2CC[C@]4([C@H]3C[C@H](C4=O)F)C | |
Source | PubChem | |
URL | https://pubchem.ncbi.nlm.nih.gov | |
Description | Data deposited in or computed by PubChem | |
Molecular Formula |
C19H27FO | |
Source | PubChem | |
URL | https://pubchem.ncbi.nlm.nih.gov | |
Description | Data deposited in or computed by PubChem | |
DSSTOX Substance ID |
DTXSID90920920 | |
Record name | Fluasterone | |
Source | EPA DSSTox | |
URL | https://comptox.epa.gov/dashboard/DTXSID90920920 | |
Description | DSSTox provides a high quality public chemistry resource for supporting improved predictive toxicology. | |
Molecular Weight |
290.4 g/mol | |
Source | PubChem | |
URL | https://pubchem.ncbi.nlm.nih.gov | |
Description | Data deposited in or computed by PubChem | |
Mechanism of Action |
Fluasterone is a synthetically stable adrenocortical steroid fluorinated analogue of dehydroepiandrosterone (DHEA), a powerful anti-inflammatory molecule with androgenic or estrogenic side effects. It is proposed that fluasterone inhibits NF-kB activation and reduces oxidative stress, but other mechanisms may play a role. Fluasterone suppresses inflammation and is effective in preclinical models of chronic inflammatory disease including psoriasis, asthma, rheumatoid arthritis, multiple sclerosis and lupus erythematosus. Fluasterone has anti-inflammatory effects inpreclinical models of chronic inflammatory disease including psoriasis, asthma, rheumatoid arthritis, multiple sclerosis and lupus erythematosus. [Aeson Pharmaceuticals Executive Report] | |
Record name | Fluasterone | |
Source | DrugBank | |
URL | https://www.drugbank.ca/drugs/DB06250 | |
Description | The DrugBank database is a unique bioinformatics and cheminformatics resource that combines detailed drug (i.e. chemical, pharmacological and pharmaceutical) data with comprehensive drug target (i.e. sequence, structure, and pathway) information. | |
Explanation | Creative Common's Attribution-NonCommercial 4.0 International License (http://creativecommons.org/licenses/by-nc/4.0/legalcode) | |
CAS No. |
112859-71-9 | |
Record name | Fluasterone | |
Source | CAS Common Chemistry | |
URL | https://commonchemistry.cas.org/detail?cas_rn=112859-71-9 | |
Description | CAS Common Chemistry is an open community resource for accessing chemical information. Nearly 500,000 chemical substances from CAS REGISTRY cover areas of community interest, including common and frequently regulated chemicals, and those relevant to high school and undergraduate chemistry classes. This chemical information, curated by our expert scientists, is provided in alignment with our mission as a division of the American Chemical Society. | |
Explanation | The data from CAS Common Chemistry is provided under a CC-BY-NC 4.0 license, unless otherwise stated. | |
Record name | Fluasterone | |
Source | ChemIDplus | |
URL | https://pubchem.ncbi.nlm.nih.gov/substance/?source=chemidplus&sourceid=0112859719 | |
Description | ChemIDplus is a free, web search system that provides access to the structure and nomenclature authority files used for the identification of chemical substances cited in National Library of Medicine (NLM) databases, including the TOXNET system. | |
Record name | Fluasterone | |
Source | DrugBank | |
URL | https://www.drugbank.ca/drugs/DB06250 | |
Description | The DrugBank database is a unique bioinformatics and cheminformatics resource that combines detailed drug (i.e. chemical, pharmacological and pharmaceutical) data with comprehensive drug target (i.e. sequence, structure, and pathway) information. | |
Explanation | Creative Common's Attribution-NonCommercial 4.0 International License (http://creativecommons.org/licenses/by-nc/4.0/legalcode) | |
Record name | Fluasterone | |
Source | EPA DSSTox | |
URL | https://comptox.epa.gov/dashboard/DTXSID90920920 | |
Description | DSSTox provides a high quality public chemistry resource for supporting improved predictive toxicology. | |
Record name | FLUASTERONE | |
Source | FDA Global Substance Registration System (GSRS) | |
URL | https://gsrs.ncats.nih.gov/ginas/app/beta/substances/R7M5UGD04G | |
Description | The FDA Global Substance Registration System (GSRS) enables the efficient and accurate exchange of information on what substances are in regulated products. Instead of relying on names, which vary across regulatory domains, countries, and regions, the GSRS knowledge base makes it possible for substances to be defined by standardized, scientific descriptions. | |
Explanation | Unless otherwise noted, the contents of the FDA website (www.fda.gov), both text and graphics, are not copyrighted. They are in the public domain and may be republished, reprinted and otherwise used freely by anyone without the need to obtain permission from FDA. Credit to the U.S. Food and Drug Administration as the source is appreciated but not required. | |
Retrosynthesis Analysis
AI-Powered Synthesis Planning: Our tool employs the Template_relevance Pistachio, Template_relevance Bkms_metabolic, Template_relevance Pistachio_ringbreaker, Template_relevance Reaxys, Template_relevance Reaxys_biocatalysis model, leveraging a vast database of chemical reactions to predict feasible synthetic routes.
One-Step Synthesis Focus: Specifically designed for one-step synthesis, it provides concise and direct routes for your target compounds, streamlining the synthesis process.
Accurate Predictions: Utilizing the extensive PISTACHIO, BKMS_METABOLIC, PISTACHIO_RINGBREAKER, REAXYS, REAXYS_BIOCATALYSIS database, our tool offers high-accuracy predictions, reflecting the latest in chemical research and data.
Strategy Settings
Precursor scoring | Relevance Heuristic |
---|---|
Min. plausibility | 0.01 |
Model | Template_relevance |
Template Set | Pistachio/Bkms_metabolic/Pistachio_ringbreaker/Reaxys/Reaxys_biocatalysis |
Top-N result to add to graph | 6 |
Feasible Synthetic Routes
体外研究产品的免责声明和信息
请注意,BenchChem 上展示的所有文章和产品信息仅供信息参考。 BenchChem 上可购买的产品专为体外研究设计,这些研究在生物体外进行。体外研究,源自拉丁语 "in glass",涉及在受控实验室环境中使用细胞或组织进行的实验。重要的是要注意,这些产品没有被归类为药物或药品,他们没有得到 FDA 的批准,用于预防、治疗或治愈任何医疗状况、疾病或疾病。我们必须强调,将这些产品以任何形式引入人类或动物的身体都是法律严格禁止的。遵守这些指南对确保研究和实验的法律和道德标准的符合性至关重要。