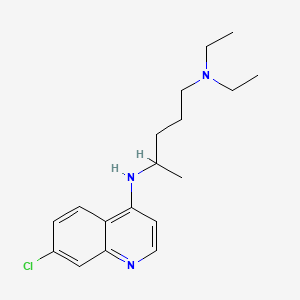
Chloroquine
概述
描述
Chloroquine is a synthetic drug primarily used in the treatment and prevention of malaria. Discovered in 1934 and introduced into medicine in the 1940s, this compound is a member of the quinoline derivatives, a series of chemically related antimalarial agents . It is effective against susceptible strains of the malarial parasites Plasmodium vivax, Plasmodium ovale, and Plasmodium falciparum, as well as certain parasitic worms and amoebas . Additionally, this compound is used in the treatment of inflammatory rheumatic diseases such as lupus erythematosus and rheumatoid arthritis .
准备方法
合成路线和反应条件
氯喹的合成涉及 4,7-二氯喹啉与 2-氨基-5-二乙基氨基戊烷的缩合反应 。该反应通过以下步骤进行:
缩合反应: 4,7-二氯喹啉与 2-氨基-5-二乙基氨基戊烷反应生成氯喹。
碱化萃取: 反应混合物进行碱化萃取以分离氯喹。
浓缩和结晶: 分离的氯喹被浓缩并结晶以提高纯度。
成盐: 纯化的氯喹然后与磷酸成盐以生产磷酸氯喹.
工业生产方法
氯喹的工业生产遵循类似的合成路线,但规模更大。该过程包括:
批量合成: 使用大量 4,7-二氯喹啉和 2-氨基-5-二乙基氨基戊烷。
连续萃取和结晶: 反应混合物进行连续萃取和结晶,以确保高产率和纯度。
质量控制: 最终产品经过严格的质量控制措施,以确保符合药品标准.
化学反应分析
反应类型
氯喹会发生各种化学反应,包括:
氧化: 氯喹可以被氧化生成喹啉衍生物。
还原: 还原反应可以改变喹啉环结构。
取代: 取代反应可以在氯和氨基上发生.
常见试剂和条件
氧化: 常见的氧化剂包括高锰酸钾和过氧化氢。
还原: 使用锂铝氢化物和硼氢化钠等还原剂。
取代: 取代反应通常涉及胺和硫醇等亲核试剂.
主要产物
氧化产物: 具有改性环结构的喹啉衍生物。
还原产物: 还原的喹啉化合物。
取代产物: 具有各种官能团的取代氯喹衍生物.
科学研究应用
Antimalarial Applications
Chloroquine is primarily known for its role in treating malaria caused by Plasmodium species. It is effective against this compound-sensitive strains of P. falciparum, P. vivax, P. ovale, and P. malariae. The drug functions by preventing the polymerization of heme into hemozoin, leading to toxic accumulation of free heme within the parasite .
Autoimmune Disorders
This compound has established roles in managing several autoimmune diseases, including:
- Systemic Lupus Erythematosus (SLE) : this compound is effective in treating different forms of SLE, including discoid lupus and systemic lupus erythematosus, and is particularly beneficial for pregnant patients .
- Rheumatoid Arthritis (RA) : It acts as a first-line disease-modifying antirheumatic drug (DMARD), preventing the activation of CD4+ T cells by inhibiting autoantigen presentation .
- Other Conditions : this compound has shown efficacy in treating skin diseases like lichen planus and Sjögren's syndrome .
Cancer Treatment
Recent studies indicate that this compound may enhance the efficacy of chemotherapy and radiation therapy in cancer treatment:
- Mechanism : this compound disrupts autophagy in cancer cells, potentially making them more susceptible to anticancer agents .
- Clinical Trials : Investigational studies have explored its use in various cancers, including local metastatic melanoma and chronic lymphocytic leukemia .
Infectious Diseases Beyond Malaria
This compound's antiviral properties have been investigated in the context of several viral infections:
- COVID-19 : Initially proposed as a treatment due to its immunomodulatory effects and ability to alter endosomal pH, this compound was used during the early stages of the COVID-19 pandemic. However, clinical trials yielded mixed results regarding its efficacy against SARS-CoV-2 .
- SARS and AIDS : Research has also explored this compound's potential against severe acute respiratory syndrome (SARS) and human immunodeficiency virus (HIV) due to its ability to inhibit viral entry .
Pharmacokinetics and Safety Profile
This compound is generally well-tolerated but can cause side effects such as retinopathy with long-term use. Monitoring is essential for patients on prolonged therapy . The safety profile varies across populations, necessitating further research to establish comprehensive guidelines for its use.
Table 1: Approved Indications for this compound
Condition | FDA Approval Status |
---|---|
Malaria | Approved |
Systemic Lupus Erythematosus | Approved |
Rheumatoid Arthritis | Approved |
Extraintestinal Amebiasis | Approved |
Cancer (adjunct therapy) | Investigational |
Table 2: Summary of Clinical Trials on this compound for COVID-19
Study Reference | Sample Size | Outcome Measure | Results Summary |
---|---|---|---|
Gao et al. | 100 | Symptom alleviation | Reported improvement but lacked peer review |
Million et al. | Various | Meta-analysis | Criticized for flawed methodologies |
Karolyi et al. | Various | Time to negative test conversion | Moderate reduction observed |
作用机制
相似化合物的比较
生物活性
Chloroquine (CQ) is a 4-aminoquinoline compound primarily known for its antimalarial properties. Its biological activity extends beyond malaria treatment, encompassing antiviral and anticancer effects. This article delves into the mechanisms, clinical applications, and research findings related to this compound's biological activity.
This compound exerts its biological effects through several mechanisms:
- Antimalarial Activity : CQ inhibits heme polymerase in Plasmodium species, leading to the accumulation of toxic heme within the parasite. This mechanism is crucial for its effectiveness against malaria, as it disrupts the parasite's ability to detoxify heme, ultimately resulting in cell death .
- Antiviral Effects : CQ has been shown to interfere with viral entry and replication. It raises the pH in endosomes, which prevents virus particles from fusing with host cell membranes. Additionally, it inhibits glycosylation of the ACE2 receptor, which is essential for SARS-CoV-2 entry into cells .
- Anticancer Properties : The compound has demonstrated immunomodulatory effects and the ability to inhibit autophagy, which can contribute to tumor growth suppression. These properties suggest potential applications in cancer therapy .
Pharmacokinetics
This compound is well-absorbed when taken orally, with bioavailability ranging from 52% to 114% depending on the formulation. The drug has a long half-life of approximately 20-60 days, allowing for sustained therapeutic effects .
Antimalarial Use
This compound remains a first-line treatment for malaria in many regions. Its efficacy against both this compound-sensitive and resistant strains of Plasmodium has been documented extensively. A notable study indicated that CQ effectively reduced parasitemia in patients with malaria, demonstrating a significant clinical response .
COVID-19 Research
This compound gained attention during the COVID-19 pandemic as a potential treatment. Several studies have investigated its efficacy:
- A multicenter observational study involving 197 patients showed that CQ treatment resulted in a median reduction of 6 days to achieve undetectable viral RNA compared to controls. Additionally, the duration of fever was significantly shorter in the CQ group .
- Another study reported that patients treated with CQ experienced lung clearance improvements on CT scans compared to those receiving standard care (lopinavir/ritonavir) .
Summary of Findings
The following table summarizes key findings from various studies on this compound:
属性
IUPAC Name |
4-N-(7-chloroquinolin-4-yl)-1-N,1-N-diethylpentane-1,4-diamine | |
---|---|---|
Source | PubChem | |
URL | https://pubchem.ncbi.nlm.nih.gov | |
Description | Data deposited in or computed by PubChem | |
InChI |
InChI=1S/C18H26ClN3/c1-4-22(5-2)12-6-7-14(3)21-17-10-11-20-18-13-15(19)8-9-16(17)18/h8-11,13-14H,4-7,12H2,1-3H3,(H,20,21) | |
Source | PubChem | |
URL | https://pubchem.ncbi.nlm.nih.gov | |
Description | Data deposited in or computed by PubChem | |
InChI Key |
WHTVZRBIWZFKQO-UHFFFAOYSA-N | |
Source | PubChem | |
URL | https://pubchem.ncbi.nlm.nih.gov | |
Description | Data deposited in or computed by PubChem | |
Canonical SMILES |
CCN(CC)CCCC(C)NC1=C2C=CC(=CC2=NC=C1)Cl | |
Source | PubChem | |
URL | https://pubchem.ncbi.nlm.nih.gov | |
Description | Data deposited in or computed by PubChem | |
Molecular Formula |
C18H26ClN3 | |
Source | PubChem | |
URL | https://pubchem.ncbi.nlm.nih.gov | |
Description | Data deposited in or computed by PubChem | |
DSSTOX Substance ID |
DTXSID2040446 | |
Record name | Chloroquine | |
Source | EPA DSSTox | |
URL | https://comptox.epa.gov/dashboard/DTXSID2040446 | |
Description | DSSTox provides a high quality public chemistry resource for supporting improved predictive toxicology. | |
Molecular Weight |
319.9 g/mol | |
Source | PubChem | |
URL | https://pubchem.ncbi.nlm.nih.gov | |
Description | Data deposited in or computed by PubChem | |
Physical Description |
Solid | |
Record name | Chloroquine | |
Source | Human Metabolome Database (HMDB) | |
URL | http://www.hmdb.ca/metabolites/HMDB0014746 | |
Description | The Human Metabolome Database (HMDB) is a freely available electronic database containing detailed information about small molecule metabolites found in the human body. | |
Explanation | HMDB is offered to the public as a freely available resource. Use and re-distribution of the data, in whole or in part, for commercial purposes requires explicit permission of the authors and explicit acknowledgment of the source material (HMDB) and the original publication (see the HMDB citing page). We ask that users who download significant portions of the database cite the HMDB paper in any resulting publications. | |
Solubility |
Bitter colorless crystals, dimorphic. Freely soluble in water, less sol in neutral or alkaline pH. Stable to heat in soln pH4 to 6.5. Practically in soluble in alcohol, benzene and chloroform /Diphosphate/, WHITE CRYSTALLINE POWDER; ODORLESS; BITTER TASTE; FREELY SOL IN WATER;PRACTICALLY INSOL IN ALCOHOL, CHLOROFORM, ETHER; AQ SOLN HAS PH OF ABOUT 4.5; PKA1= 7; PKA2= 9.2 /PHOSPHATE/, VERY SLIGHTLY SOL IN WATER; SOL IN DIL ACIDS, CHLOROFORM, ETHER, Insoluble in alcohol, benzene, chloroform, ether., 1.75e-02 g/L | |
Record name | CHLOROQUINE | |
Source | Hazardous Substances Data Bank (HSDB) | |
URL | https://pubchem.ncbi.nlm.nih.gov/source/hsdb/3029 | |
Description | The Hazardous Substances Data Bank (HSDB) is a toxicology database that focuses on the toxicology of potentially hazardous chemicals. It provides information on human exposure, industrial hygiene, emergency handling procedures, environmental fate, regulatory requirements, nanomaterials, and related areas. The information in HSDB has been assessed by a Scientific Review Panel. | |
Record name | Chloroquine | |
Source | Human Metabolome Database (HMDB) | |
URL | http://www.hmdb.ca/metabolites/HMDB0014746 | |
Description | The Human Metabolome Database (HMDB) is a freely available electronic database containing detailed information about small molecule metabolites found in the human body. | |
Explanation | HMDB is offered to the public as a freely available resource. Use and re-distribution of the data, in whole or in part, for commercial purposes requires explicit permission of the authors and explicit acknowledgment of the source material (HMDB) and the original publication (see the HMDB citing page). We ask that users who download significant portions of the database cite the HMDB paper in any resulting publications. | |
Mechanism of Action |
Chloroquine inhibits the action of heme polymerase in malarial trophozoites, preventing the conversion of heme to hemazoin. _Plasmodium_ species continue to accumulate toxic heme, killing the parasite. Chloroquine passively diffuses through cell membranes and into endosomes, lysosomes, and Golgi vesicles; where it becomes protonated, trapping the chloroquine in the organelle and raising the surrounding pH. The raised pH in endosomes, prevent virus particles from utilizing their activity for fusion and entry into the cell. Chloroquine does not affect the level of ACE2 expression on cell surfaces, but inhibits terminal glycosylation of ACE2, the receptor that SARS-CoV and SARS-CoV-2 target for cell entry. ACE2 that is not in the glycosylated state may less efficiently interact with the SARS-CoV-2 spike protein, further inhibiting viral entry., The exact mechanism of antimalarial activity of chloroquine has not been determined. The 4-aminoquinoline derivatives appear to bind to nucleoproteins and interfere with protein synthesis in susceptible organisms; the drugs intercalate readily into double-stranded DNA and inhibit both DNA and RNA polymerase. In addition, studies using chloroquine indicate that the drug apparently concentrates in parasite digestive vacuoles, increases the pH of the vacuoles, and interferes with the parasite's ability to metabolize and utilize erythrocyte hemoglobin. Plasmodial forms that do not have digestive vacuoles and do not utilize hemoglobin, such as exoerythrocytic forms, are not affected by chloroquine., The 4-aminoquinoline derivatives, including chloroquine, also have anti-inflammatory activity; however, the mechanism(s) of action of the drugs in the treatment of rheumatoid arthritis and lupus erythematosus has not been determined. Chloroquine reportedly antagonizes histamine in vitro, has antiserotonin effects, and inhibits prostaglandin effects in mammalian cells presumably by inhibiting conversion of arachidonic acid to prostaglandin F2. In vitro studies indicate that chloroquine also inhibits chemotaxis of polymorphonuclear leukocytes, macrophages, and eosinophils., Antiprotozoal-Malaria: /Mechanism of action/ may be based on ability of chloroquine to bind and alter the properties of DNA. Chloroquine also is taken up into the acidic food vacuoles of the parasite in the erythrocyte. It increases the pH of the acid vesicles, interfering with vesicle functions and possibly inhibiting phospholipid metabolism. In suppressive treatment, chloroquine inhibits the erythrocytic stage of development of plasmodia. In acute attacks of malaria, chloroquine interrupts erythrocytic schizogony of the parasite. its ability to concentrate in parasitized erythrocytes may account for its selective toxicity against the erythrocytic stages of plasmodial infection., Antirheumatic-Chloroquine is though to act as a mild immunosuppressant, inhibiting the production of rheumatoid factor and acute phase reactants. It also accumulates in white blood cells, stabilizing lysosomal membranes and inhibiting the activity of many enzymes, including collagenase and the proteases that cause cartilage breakdown. | |
Record name | Chloroquine | |
Source | DrugBank | |
URL | https://www.drugbank.ca/drugs/DB00608 | |
Description | The DrugBank database is a unique bioinformatics and cheminformatics resource that combines detailed drug (i.e. chemical, pharmacological and pharmaceutical) data with comprehensive drug target (i.e. sequence, structure, and pathway) information. | |
Explanation | Creative Common's Attribution-NonCommercial 4.0 International License (http://creativecommons.org/licenses/by-nc/4.0/legalcode) | |
Record name | CHLOROQUINE | |
Source | Hazardous Substances Data Bank (HSDB) | |
URL | https://pubchem.ncbi.nlm.nih.gov/source/hsdb/3029 | |
Description | The Hazardous Substances Data Bank (HSDB) is a toxicology database that focuses on the toxicology of potentially hazardous chemicals. It provides information on human exposure, industrial hygiene, emergency handling procedures, environmental fate, regulatory requirements, nanomaterials, and related areas. The information in HSDB has been assessed by a Scientific Review Panel. | |
Color/Form |
WHITE TO SLIGHTLY YELLOW, CRYSTALLINE POWDER, Colorless crystals | |
CAS No. |
54-05-7 | |
Record name | Chloroquine | |
Source | CAS Common Chemistry | |
URL | https://commonchemistry.cas.org/detail?cas_rn=54-05-7 | |
Description | CAS Common Chemistry is an open community resource for accessing chemical information. Nearly 500,000 chemical substances from CAS REGISTRY cover areas of community interest, including common and frequently regulated chemicals, and those relevant to high school and undergraduate chemistry classes. This chemical information, curated by our expert scientists, is provided in alignment with our mission as a division of the American Chemical Society. | |
Explanation | The data from CAS Common Chemistry is provided under a CC-BY-NC 4.0 license, unless otherwise stated. | |
Record name | Chloroquine [USP:INN:BAN] | |
Source | ChemIDplus | |
URL | https://pubchem.ncbi.nlm.nih.gov/substance/?source=chemidplus&sourceid=0000054057 | |
Description | ChemIDplus is a free, web search system that provides access to the structure and nomenclature authority files used for the identification of chemical substances cited in National Library of Medicine (NLM) databases, including the TOXNET system. | |
Record name | Chloroquine | |
Source | DrugBank | |
URL | https://www.drugbank.ca/drugs/DB00608 | |
Description | The DrugBank database is a unique bioinformatics and cheminformatics resource that combines detailed drug (i.e. chemical, pharmacological and pharmaceutical) data with comprehensive drug target (i.e. sequence, structure, and pathway) information. | |
Explanation | Creative Common's Attribution-NonCommercial 4.0 International License (http://creativecommons.org/licenses/by-nc/4.0/legalcode) | |
Record name | chloroquine | |
Source | DTP/NCI | |
URL | https://dtp.cancer.gov/dtpstandard/servlet/dwindex?searchtype=NSC&outputformat=html&searchlist=187208 | |
Description | The NCI Development Therapeutics Program (DTP) provides services and resources to the academic and private-sector research communities worldwide to facilitate the discovery and development of new cancer therapeutic agents. | |
Explanation | Unless otherwise indicated, all text within NCI products is free of copyright and may be reused without our permission. Credit the National Cancer Institute as the source. | |
Record name | Chloroquine | |
Source | EPA DSSTox | |
URL | https://comptox.epa.gov/dashboard/DTXSID2040446 | |
Description | DSSTox provides a high quality public chemistry resource for supporting improved predictive toxicology. | |
Record name | Chloroquine | |
Source | European Chemicals Agency (ECHA) | |
URL | https://echa.europa.eu/substance-information/-/substanceinfo/100.000.175 | |
Description | The European Chemicals Agency (ECHA) is an agency of the European Union which is the driving force among regulatory authorities in implementing the EU's groundbreaking chemicals legislation for the benefit of human health and the environment as well as for innovation and competitiveness. | |
Explanation | Use of the information, documents and data from the ECHA website is subject to the terms and conditions of this Legal Notice, and subject to other binding limitations provided for under applicable law, the information, documents and data made available on the ECHA website may be reproduced, distributed and/or used, totally or in part, for non-commercial purposes provided that ECHA is acknowledged as the source: "Source: European Chemicals Agency, http://echa.europa.eu/". Such acknowledgement must be included in each copy of the material. ECHA permits and encourages organisations and individuals to create links to the ECHA website under the following cumulative conditions: Links can only be made to webpages that provide a link to the Legal Notice page. | |
Record name | CHLOROQUINE | |
Source | FDA Global Substance Registration System (GSRS) | |
URL | https://gsrs.ncats.nih.gov/ginas/app/beta/substances/886U3H6UFF | |
Description | The FDA Global Substance Registration System (GSRS) enables the efficient and accurate exchange of information on what substances are in regulated products. Instead of relying on names, which vary across regulatory domains, countries, and regions, the GSRS knowledge base makes it possible for substances to be defined by standardized, scientific descriptions. | |
Explanation | Unless otherwise noted, the contents of the FDA website (www.fda.gov), both text and graphics, are not copyrighted. They are in the public domain and may be republished, reprinted and otherwise used freely by anyone without the need to obtain permission from FDA. Credit to the U.S. Food and Drug Administration as the source is appreciated but not required. | |
Record name | CHLOROQUINE | |
Source | Hazardous Substances Data Bank (HSDB) | |
URL | https://pubchem.ncbi.nlm.nih.gov/source/hsdb/3029 | |
Description | The Hazardous Substances Data Bank (HSDB) is a toxicology database that focuses on the toxicology of potentially hazardous chemicals. It provides information on human exposure, industrial hygiene, emergency handling procedures, environmental fate, regulatory requirements, nanomaterials, and related areas. The information in HSDB has been assessed by a Scientific Review Panel. | |
Record name | Chloroquine | |
Source | Human Metabolome Database (HMDB) | |
URL | http://www.hmdb.ca/metabolites/HMDB0014746 | |
Description | The Human Metabolome Database (HMDB) is a freely available electronic database containing detailed information about small molecule metabolites found in the human body. | |
Explanation | HMDB is offered to the public as a freely available resource. Use and re-distribution of the data, in whole or in part, for commercial purposes requires explicit permission of the authors and explicit acknowledgment of the source material (HMDB) and the original publication (see the HMDB citing page). We ask that users who download significant portions of the database cite the HMDB paper in any resulting publications. | |
Melting Point |
87-89.5, 87 °C, 289 °C | |
Record name | Chloroquine | |
Source | DrugBank | |
URL | https://www.drugbank.ca/drugs/DB00608 | |
Description | The DrugBank database is a unique bioinformatics and cheminformatics resource that combines detailed drug (i.e. chemical, pharmacological and pharmaceutical) data with comprehensive drug target (i.e. sequence, structure, and pathway) information. | |
Explanation | Creative Common's Attribution-NonCommercial 4.0 International License (http://creativecommons.org/licenses/by-nc/4.0/legalcode) | |
Record name | CHLOROQUINE | |
Source | Hazardous Substances Data Bank (HSDB) | |
URL | https://pubchem.ncbi.nlm.nih.gov/source/hsdb/3029 | |
Description | The Hazardous Substances Data Bank (HSDB) is a toxicology database that focuses on the toxicology of potentially hazardous chemicals. It provides information on human exposure, industrial hygiene, emergency handling procedures, environmental fate, regulatory requirements, nanomaterials, and related areas. The information in HSDB has been assessed by a Scientific Review Panel. | |
Record name | Chloroquine | |
Source | Human Metabolome Database (HMDB) | |
URL | http://www.hmdb.ca/metabolites/HMDB0014746 | |
Description | The Human Metabolome Database (HMDB) is a freely available electronic database containing detailed information about small molecule metabolites found in the human body. | |
Explanation | HMDB is offered to the public as a freely available resource. Use and re-distribution of the data, in whole or in part, for commercial purposes requires explicit permission of the authors and explicit acknowledgment of the source material (HMDB) and the original publication (see the HMDB citing page). We ask that users who download significant portions of the database cite the HMDB paper in any resulting publications. | |
Synthesis routes and methods I
Procedure details
Synthesis routes and methods II
Procedure details
Retrosynthesis Analysis
AI-Powered Synthesis Planning: Our tool employs the Template_relevance Pistachio, Template_relevance Bkms_metabolic, Template_relevance Pistachio_ringbreaker, Template_relevance Reaxys, Template_relevance Reaxys_biocatalysis model, leveraging a vast database of chemical reactions to predict feasible synthetic routes.
One-Step Synthesis Focus: Specifically designed for one-step synthesis, it provides concise and direct routes for your target compounds, streamlining the synthesis process.
Accurate Predictions: Utilizing the extensive PISTACHIO, BKMS_METABOLIC, PISTACHIO_RINGBREAKER, REAXYS, REAXYS_BIOCATALYSIS database, our tool offers high-accuracy predictions, reflecting the latest in chemical research and data.
Strategy Settings
Precursor scoring | Relevance Heuristic |
---|---|
Min. plausibility | 0.01 |
Model | Template_relevance |
Template Set | Pistachio/Bkms_metabolic/Pistachio_ringbreaker/Reaxys/Reaxys_biocatalysis |
Top-N result to add to graph | 6 |
Feasible Synthetic Routes
体外研究产品的免责声明和信息
请注意,BenchChem 上展示的所有文章和产品信息仅供信息参考。 BenchChem 上可购买的产品专为体外研究设计,这些研究在生物体外进行。体外研究,源自拉丁语 "in glass",涉及在受控实验室环境中使用细胞或组织进行的实验。重要的是要注意,这些产品没有被归类为药物或药品,他们没有得到 FDA 的批准,用于预防、治疗或治愈任何医疗状况、疾病或疾病。我们必须强调,将这些产品以任何形式引入人类或动物的身体都是法律严格禁止的。遵守这些指南对确保研究和实验的法律和道德标准的符合性至关重要。