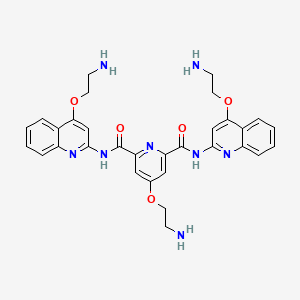
Pyridostatin
描述
Synthesis Analysis
The synthesis of Pyridostatin involves a series of G-quadruplex interacting small molecules based on a N, N′-bis (quinolinyl)pyridine-2,6-dicarboxamide scaffold . The synthetic analogues were evaluated for their ability to stabilize telomeric G-quadruplex DNA, some of which showed very high stabilization potential associated with high selectivity over double-stranded DNA .Molecular Structure Analysis
The molecular structure of Pyridostatin is based on a N, N′-bis (quinolinyl)pyridine-2,6-dicarboxamide scaffold . The NMR solution structure of the 1:1 complex of a pyridostatin derivative bound to a G-quadruplex has been described .Chemical Reactions Analysis
Pyridostatin is a G-quadruplex DNA stabilizing agent . It interacts with the G-quadruplex DNA and stabilizes it .Physical And Chemical Properties Analysis
Pyridostatin has a molecular formula of C31H32N8O5 and a molecular weight of 596.64 .科学研究应用
Structural Analysis of G-Quadruplexes
Pyridostatin has been instrumental in the structural elucidation of G-quadruplexes. Researchers have utilized PDS to stabilize G4 structures, allowing for detailed studies using techniques like NMR spectroscopy and X-ray crystallography . This has advanced our understanding of the structural basis of G4 binding and the specificity of ligands like PDS.
Cancer Research and Therapy
PDS is known to target G-quadruplexes present in oncogenes, making it a potential therapeutic agent. It has been shown to promote the cytotoxicity of platinum-based compounds in cancer cells by targeting proteins such as SUB1, which is involved in DNA repair mechanisms . This suggests that PDS could be used to enhance the efficacy of existing cancer treatments.
Gene Expression Modulation
The ability of PDS to induce and stabilize G-quadruplexes affects gene expression. It has been observed to downregulate certain proteins while upregulating others in HeLa cancer cells, indicating a complex role in the regulation of gene expression . This property can be harnessed to study gene function and regulation.
Molecular Biology Techniques
In molecular biology, PDS can be used in assays to study the formation and stability of G-quadruplexes. For instance, native PAGE assays have been conducted with PDS to analyze the interaction between ligands and G4 DNA . This helps in understanding the dynamics of G4 formation and their biological significance.
Biomarker Identification
Due to its specificity for G-quadruplexes, PDS can aid in identifying biomarkers for diseases like cancer. G4 structures are prevalent in regulatory regions of genes associated with cancer, and PDS can be used to pinpoint these sites for diagnostic purposes .
Drug Design and Development
The interaction of PDS with G-quadruplexes offers insights into the design of novel drugs. By understanding how PDS binds to G4s, researchers can develop new compounds that mimic its action or use it as a scaffold for creating drugs with improved specificity and efficacy .
作用机制
Target of Action
Pyridostatin (PDS) is a well-known G-quadruplex (G4) inducer and stabilizer . Its primary targets are the G4 structures in the DNA, which are formed by hydrogen bonding of guanine bases in G-rich DNA or RNA sequences . These structures are found in over 10,000 potential sequences in human chromatin, especially in highly transcribed genes and regulatory regions . PDS also targets the SUB1 gene, which encodes the human positive cofactor and DNA lesion sensor PC4 .
Mode of Action
PDS acts mainly on nucleotide chains and can sustain the loading forces produced by DNA and RNA polymerases . As a result, these polymerases cannot open G4s, leading to DNA double-strand breaks (DSBs) . PDS also downregulates the expression of PC4, inhibiting the repair of DNA lesions .
Biochemical Pathways
PDS significantly downregulates 22 proteins but upregulates 16 proteins in HeLa cancer cells . The downregulated proteins consequently upregulate 6 proteins to activate cyclin and cell cycle regulation . This suggests that PDS regulation on gene expression is far more complicated than inducing/stabilizing G4 structures .
Pharmacokinetics
The structural features of pds, including a potentially planar electron-rich aromatic surface and the ability to participate in hydrogen bonding, suggest that it may have good bioavailability . The rotatable bonds in PDS provide a degree of flexibility, which could influence its absorption, distribution, metabolism, and excretion .
Result of Action
The action of PDS leads to a number of molecular and cellular effects. It can effectively regulate G4 functions, directly damage G4 structures, and activate multiple antitumor signaling pathways . The downregulation of PC4 dramatically promotes the cytotoxicity of transplatin toward HeLa cells, contributing to the retardation of the repair of DNA lesions mediated by PC4 .
Action Environment
The action, efficacy, and stability of PDS can be influenced by various environmental factors. For instance, the presence of large amounts of dsDNA can affect the specificity of PDS for covalent binding to G4 domains
安全和危害
Pyridostatin is used for research purposes only and is not intended for human, veterinary diagnostic or therapeutic use . It is recommended to handle it with care and follow safety guidelines provided in the safety data sheet .
Relevant Papers Several papers have been published on Pyridostatin, discussing its role in antiviral therapy , its interaction with G-quadruplex DNA , and its potential in promoting DNA damage . These papers provide valuable insights into the properties and potential applications of Pyridostatin.
属性
IUPAC Name |
4-(2-aminoethoxy)-2-N,6-N-bis[4-(2-aminoethoxy)quinolin-2-yl]pyridine-2,6-dicarboxamide | |
---|---|---|
Source | PubChem | |
URL | https://pubchem.ncbi.nlm.nih.gov | |
Description | Data deposited in or computed by PubChem | |
InChI |
InChI=1S/C31H32N8O5/c32-9-12-42-19-15-24(30(40)38-28-17-26(43-13-10-33)20-5-1-3-7-22(20)36-28)35-25(16-19)31(41)39-29-18-27(44-14-11-34)21-6-2-4-8-23(21)37-29/h1-8,15-18H,9-14,32-34H2,(H,36,38,40)(H,37,39,41) | |
Source | PubChem | |
URL | https://pubchem.ncbi.nlm.nih.gov | |
Description | Data deposited in or computed by PubChem | |
InChI Key |
VGHSATQVJCTKEF-UHFFFAOYSA-N | |
Source | PubChem | |
URL | https://pubchem.ncbi.nlm.nih.gov | |
Description | Data deposited in or computed by PubChem | |
Canonical SMILES |
C1=CC=C2C(=C1)C(=CC(=N2)NC(=O)C3=CC(=CC(=N3)C(=O)NC4=NC5=CC=CC=C5C(=C4)OCCN)OCCN)OCCN | |
Source | PubChem | |
URL | https://pubchem.ncbi.nlm.nih.gov | |
Description | Data deposited in or computed by PubChem | |
Molecular Formula |
C31H32N8O5 | |
Source | PubChem | |
URL | https://pubchem.ncbi.nlm.nih.gov | |
Description | Data deposited in or computed by PubChem | |
Molecular Weight |
596.6 g/mol | |
Source | PubChem | |
URL | https://pubchem.ncbi.nlm.nih.gov | |
Description | Data deposited in or computed by PubChem | |
Product Name |
Pyridostatin |
Retrosynthesis Analysis
AI-Powered Synthesis Planning: Our tool employs the Template_relevance Pistachio, Template_relevance Bkms_metabolic, Template_relevance Pistachio_ringbreaker, Template_relevance Reaxys, Template_relevance Reaxys_biocatalysis model, leveraging a vast database of chemical reactions to predict feasible synthetic routes.
One-Step Synthesis Focus: Specifically designed for one-step synthesis, it provides concise and direct routes for your target compounds, streamlining the synthesis process.
Accurate Predictions: Utilizing the extensive PISTACHIO, BKMS_METABOLIC, PISTACHIO_RINGBREAKER, REAXYS, REAXYS_BIOCATALYSIS database, our tool offers high-accuracy predictions, reflecting the latest in chemical research and data.
Strategy Settings
Precursor scoring | Relevance Heuristic |
---|---|
Min. plausibility | 0.01 |
Model | Template_relevance |
Template Set | Pistachio/Bkms_metabolic/Pistachio_ringbreaker/Reaxys/Reaxys_biocatalysis |
Top-N result to add to graph | 6 |
Feasible Synthetic Routes
Q & A
Q1: What is the primary molecular target of Pyridostatin?
A1: Pyridostatin exhibits high specificity for G-quadruplexes (G4s), a non-canonical secondary structure formed by guanine-rich DNA or RNA sequences. [, , , , , , , , , , , , ]
Q2: How does Pyridostatin interact with G-quadruplexes?
A2: Pyridostatin binds to G4s through a combination of π-π stacking interactions between its aromatic rings and the guanine tetrads, as well as hydrogen bonding and electrostatic interactions between its aliphatic amine side chains and the phosphate backbone of the DNA or RNA. [, ] This binding stabilizes the G4 structure, interfering with its normal biological functions. [, , , ]
Q3: What are the downstream consequences of Pyridostatin binding to G-quadruplexes in cells?
A3: Pyridostatin binding to G4s can disrupt a variety of cellular processes, depending on the location and function of the targeted G4. These effects include:
- Inhibition of Telomerase Activity: Pyridostatin can bind to telomeric G4s, inhibiting the activity of telomerase, an enzyme essential for maintaining telomere length in cancer cells. [, , , ]
- Induction of DNA Damage: By interfering with DNA replication and repair processes, Pyridostatin can induce DNA double-strand breaks (DSBs), leading to genomic instability and cell death. [, , , , , , , , ]
- Modulation of Gene Expression: Pyridostatin can influence gene expression by binding to G4s located in promoter regions or within gene bodies, affecting transcription and potentially leading to changes in protein levels. [, , , , ]
- Inhibition of Viral Replication: Recent research suggests that Pyridostatin can inhibit the replication of certain viruses, such as rhinoviruses and enteroviruses, by targeting G4s within their RNA genomes. [, ]
Q4: How does Pyridostatin affect the innate immune response in cancer cells?
A4: Pyridostatin has been shown to induce the formation of micronuclei, fragments of chromosomes not incorporated into daughter cells during cell division. These micronuclei can then trigger the cGAS/STING pathway, a key component of the innate immune response, leading to the production of type I interferons and activation of other immune-related genes. [, , ]
Q5: What is the molecular formula and weight of Pyridostatin?
A5: The molecular formula for Pyridostatin is C26H27N7O2, and its molecular weight is 473.55 g/mol.
Q6: Is there spectroscopic data available for Pyridostatin?
A6: Yes, various spectroscopic techniques have been used to characterize Pyridostatin and its interactions with G4s. These include:
- Nuclear Magnetic Resonance (NMR) Spectroscopy: Used to determine the structure of Pyridostatin bound to G4s. []
- Circular Dichroism (CD) Spectroscopy: Employed to study the conformational changes induced in G4s upon Pyridostatin binding. [, ]
- Fluorescence Spectroscopy: Used to investigate the binding affinity and stoichiometry of Pyridostatin with G4s. [, ]
- Ultraviolet Resonance Raman (UVRR) Spectroscopy: Provides detailed information about the specific chemical groups involved in the interaction between Pyridostatin and G4s. []
Q7: Have computational methods been employed to study Pyridostatin and its interactions with G-quadruplexes?
A7: Yes, computational chemistry and modeling techniques have played a significant role in understanding the binding mode, affinity, and selectivity of Pyridostatin towards G4s. These methods include:
- Molecular Docking: Used to predict the binding pose and interactions of Pyridostatin within the G4 structure. [, ]
- Molecular Dynamics (MD) Simulations: Provide insights into the dynamic behavior of Pyridostatin-G4 complexes and the stability of these interactions over time. []
- Quantitative Structure-Activity Relationship (QSAR) Studies: Used to correlate the structural features of Pyridostatin and its analogs with their biological activity, providing guidance for the design of more potent and selective G4 binders. [, ]
Q8: How do structural modifications of Pyridostatin impact its activity and selectivity?
A8: SAR studies have been instrumental in understanding the key structural features of Pyridostatin that contribute to its G4 binding affinity and selectivity.
- Aromatic Core: The central pyridine ring and the two quinoline rings are essential for π-π stacking interactions with the G-tetrads. Modifications to these rings can significantly impact binding affinity. [, , ]
- Linker Length and Flexibility: The length and flexibility of the linkers connecting the aromatic rings to the central pyridine can affect the ability of Pyridostatin to adapt to different G4 conformations. Optimal linker length and flexibility are crucial for achieving high affinity and selectivity. [, , ]
- Side Chain Substituents: The amine side chains contribute to binding affinity through electrostatic interactions and hydrogen bonding with the DNA or RNA backbone. Modifications to these side chains can modulate the binding affinity and selectivity of Pyridostatin analogs. [, ]
Q9: What is known about the ADME properties of Pyridostatin?
A9: While detailed PK/PD studies are still limited, some research has provided insights into the ADME properties of Pyridostatin:
- Distribution: Pyridostatin has been shown to effectively target genomic loci associated with G4 binding proteins in cells, indicating successful cellular uptake and distribution. []
Q10: What evidence supports the efficacy of Pyridostatin in preclinical models?
A10: Preclinical studies have demonstrated promising anticancer activity of Pyridostatin in various in vitro and in vivo models:
- In Vitro Studies: Pyridostatin has shown potent cytotoxicity against a range of cancer cell lines, including those deficient in BRCA1/2 genes, which are involved in DNA repair. [, , , , , , ]
- Animal Models: In vivo studies using mouse models of cancer have demonstrated that Pyridostatin can inhibit tumor growth and improve survival. [, ]
Q11: Are there any known mechanisms of resistance to Pyridostatin?
A11: The emergence of resistance to anticancer agents is a major challenge. While specific resistance mechanisms to Pyridostatin have not been fully elucidated, potential mechanisms may involve:
Q12: Is there evidence for cross-resistance between Pyridostatin and other anticancer agents?
A12: Cross-resistance, where resistance to one drug confers resistance to other structurally or mechanistically related compounds, is a concern in cancer treatment. Limited data is available on cross-resistance with Pyridostatin. Research suggests that cells with increased expression of the DNA helicase BRIP1, involved in G4 unwinding, show resistance to both Pyridostatin and the G4 stabilizer TMPyP4, indicating a potential for cross-resistance among G4 ligands. []
Q13: What is the safety profile of Pyridostatin?
A13: While Pyridostatin has shown promising anticancer activity, thorough safety assessments are crucial before clinical translation. Further research is needed to fully elucidate the potential toxicity and long-term safety profile of Pyridostatin.
Q14: How does Pyridostatin research highlight the importance of cross-disciplinary collaboration?
A14: The development and characterization of Pyridostatin as a potential therapeutic agent exemplify the significance of cross-disciplinary research. This endeavor involves collaborations between:
- Chemistry and Medicinal Chemistry: Synthesis and structural optimization of Pyridostatin analogs with improved potency, selectivity, and pharmacological properties. [, , ]
- Molecular Biology and Genetics: Understanding the biological roles of G4s, identifying Pyridostatin targets, and elucidating its mechanisms of action. [, , , , , , ]
- Cell Biology and Biochemistry: Investigating the cellular effects of Pyridostatin, including its impact on DNA replication, repair, transcription, and cell signaling pathways. [, , , , , , , , , ]
- Computational Chemistry and Bioinformatics: Predicting G4 formation, modeling Pyridostatin-G4 interactions, and analyzing genomic data to identify potential therapeutic targets. [, , , , , ]
- Pharmacology and Toxicology: Evaluating the absorption, distribution, metabolism, excretion, and safety profile of Pyridostatin. [, ]
体外研究产品的免责声明和信息
请注意,BenchChem 上展示的所有文章和产品信息仅供信息参考。 BenchChem 上可购买的产品专为体外研究设计,这些研究在生物体外进行。体外研究,源自拉丁语 "in glass",涉及在受控实验室环境中使用细胞或组织进行的实验。重要的是要注意,这些产品没有被归类为药物或药品,他们没有得到 FDA 的批准,用于预防、治疗或治愈任何医疗状况、疾病或疾病。我们必须强调,将这些产品以任何形式引入人类或动物的身体都是法律严格禁止的。遵守这些指南对确保研究和实验的法律和道德标准的符合性至关重要。