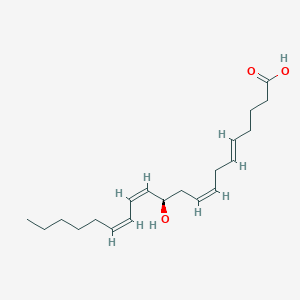
11(R)-Hete
描述
11(R)-HETE is an 11-HETE in which the chiral centre at position 11 has R-configuration. It is a conjugate acid of an this compound(1-). It is an enantiomer of an 11(S)-HETE.
This compound is a natural product found in Gersemia fruticosa with data available.
属性
IUPAC Name |
(5Z,8Z,11R,12E,14Z)-11-hydroxyicosa-5,8,12,14-tetraenoic acid | |
---|---|---|
Source | PubChem | |
URL | https://pubchem.ncbi.nlm.nih.gov | |
Description | Data deposited in or computed by PubChem | |
InChI |
InChI=1S/C20H32O3/c1-2-3-4-5-7-10-13-16-19(21)17-14-11-8-6-9-12-15-18-20(22)23/h6-7,9-11,13-14,16,19,21H,2-5,8,12,15,17-18H2,1H3,(H,22,23)/b9-6-,10-7-,14-11-,16-13+/t19-/m0/s1 | |
Source | PubChem | |
URL | https://pubchem.ncbi.nlm.nih.gov | |
Description | Data deposited in or computed by PubChem | |
InChI Key |
GCZRCCHPLVMMJE-WXMXURGXSA-N | |
Source | PubChem | |
URL | https://pubchem.ncbi.nlm.nih.gov | |
Description | Data deposited in or computed by PubChem | |
Canonical SMILES |
CCCCCC=CC=CC(CC=CCC=CCCCC(=O)O)O | |
Source | PubChem | |
URL | https://pubchem.ncbi.nlm.nih.gov | |
Description | Data deposited in or computed by PubChem | |
Isomeric SMILES |
CCCCC/C=C\C=C\[C@@H](C/C=C\C/C=C\CCCC(=O)O)O | |
Source | PubChem | |
URL | https://pubchem.ncbi.nlm.nih.gov | |
Description | Data deposited in or computed by PubChem | |
Molecular Formula |
C20H32O3 | |
Source | PubChem | |
URL | https://pubchem.ncbi.nlm.nih.gov | |
Description | Data deposited in or computed by PubChem | |
DSSTOX Substance ID |
DTXSID601318371 | |
Record name | 11(R)-HETE | |
Source | EPA DSSTox | |
URL | https://comptox.epa.gov/dashboard/DTXSID601318371 | |
Description | DSSTox provides a high quality public chemistry resource for supporting improved predictive toxicology. | |
Molecular Weight |
320.5 g/mol | |
Source | PubChem | |
URL | https://pubchem.ncbi.nlm.nih.gov | |
Description | Data deposited in or computed by PubChem | |
CAS No. |
73347-43-0 | |
Record name | 11(R)-HETE | |
Source | CAS Common Chemistry | |
URL | https://commonchemistry.cas.org/detail?cas_rn=73347-43-0 | |
Description | CAS Common Chemistry is an open community resource for accessing chemical information. Nearly 500,000 chemical substances from CAS REGISTRY cover areas of community interest, including common and frequently regulated chemicals, and those relevant to high school and undergraduate chemistry classes. This chemical information, curated by our expert scientists, is provided in alignment with our mission as a division of the American Chemical Society. | |
Explanation | The data from CAS Common Chemistry is provided under a CC-BY-NC 4.0 license, unless otherwise stated. | |
Record name | 11(R)-HETE | |
Source | EPA DSSTox | |
URL | https://comptox.epa.gov/dashboard/DTXSID601318371 | |
Description | DSSTox provides a high quality public chemistry resource for supporting improved predictive toxicology. | |
Retrosynthesis Analysis
AI-Powered Synthesis Planning: Our tool employs the Template_relevance Pistachio, Template_relevance Bkms_metabolic, Template_relevance Pistachio_ringbreaker, Template_relevance Reaxys, Template_relevance Reaxys_biocatalysis model, leveraging a vast database of chemical reactions to predict feasible synthetic routes.
One-Step Synthesis Focus: Specifically designed for one-step synthesis, it provides concise and direct routes for your target compounds, streamlining the synthesis process.
Accurate Predictions: Utilizing the extensive PISTACHIO, BKMS_METABOLIC, PISTACHIO_RINGBREAKER, REAXYS, REAXYS_BIOCATALYSIS database, our tool offers high-accuracy predictions, reflecting the latest in chemical research and data.
Strategy Settings
Precursor scoring | Relevance Heuristic |
---|---|
Min. plausibility | 0.01 |
Model | Template_relevance |
Template Set | Pistachio/Bkms_metabolic/Pistachio_ringbreaker/Reaxys/Reaxys_biocatalysis |
Top-N result to add to graph | 6 |
Feasible Synthetic Routes
Q1: What is the biosynthetic pathway of 11(R)-HETE and its metabolite, 11-oxo-ETE?
A1: this compound is generated from arachidonic acid (AA) primarily through the enzymatic activity of cyclooxygenase-2 (COX-2) [, , ]. This pathway is particularly relevant in cell types expressing high levels of COX-2, such as epithelial cells [, ]. Interestingly, this compound can be further metabolized by 15-hydroxyprostaglandin dehydrogenase (15-PGDH), an enzyme typically associated with prostaglandin metabolism, to form 11-oxo-5,8,12,14-(Z,Z,E,Z)-eicosatetraenoic acid (11-oxo-ETE) [, ].
Q2: Does 11-oxo-ETE have any biological activity?
A2: Yes, research suggests that 11-oxo-ETE acts as an antiproliferative eicosanoid, particularly in endothelial cells. Studies using human umbilical vein endothelial cells (HUVECs) revealed that 11-oxo-ETE inhibited cell proliferation with a potency similar to 15-deoxy-Δ12,14-prostaglandin J2 (15d-PGJ2), a known antiproliferative prostaglandin []. The half-maximal inhibitory concentration (IC50) of 11-oxo-ETE for HUVEC proliferation was determined to be 2.1 μM [].
Q3: How is 11-oxo-ETE further metabolized within the cell?
A3: 11-oxo-ETE readily conjugates with intracellular glutathione (GSH) through the action of glutathione S-transferase (GST) enzymes. This reaction yields the 11-oxo-ETE-GSH (OEG) adduct, which has been identified and confirmed in LoVo cells using LC-MS/MS analysis [].
Q4: Are there other oxo-ETEs produced through similar pathways?
A4: Yes, 15-oxo-5,8,11,13-(Z,Z,Z,E)-ETE (15-oxo-ETE) is another oxo-ETE generated through a comparable pathway. It arises from the metabolism of 15(S)-hydroxyeicosatetraenoic acid (15(S)-HETE) by 15-PGDH []. Notably, aspirin treatment can lead to the formation of 15(R)-HETE, which can be converted to 15-oxo-ETE by an as yet unidentified dehydrogenase [].
Q5: What analytical techniques are used to study these eicosanoids?
A5: A combination of techniques is employed to identify and quantify these eicosanoids and their metabolites. These include:
- Targeted lipidomics: This approach allows for the identification and quantification of specific lipid species, including this compound, 15(S)-HETE, 11-oxo-ETE, and 15-oxo-ETE [, ].
- Stable isotope dilution methodology: This method enhances the accuracy and precision of quantification by using isotopically labeled internal standards [].
- Chiral liquid chromatography (LC): This technique separates enantiomers, such as 15(S)-HETE and 15(R)-HETE, which is crucial for understanding their distinct biological roles [, ].
- Electron capture atmospheric pressure chemical ionization (ECAPCI)/mass spectrometry (MS) and LC-multiple reaction monitoring mass spectrometry (LC-MRM/MS): These highly sensitive and specific techniques are used for the detection and quantification of eicosanoids and their metabolites [, ].
Q6: What is the significance of studying these pathways in the context of cancer?
A6: The COX-2 enzyme is often overexpressed in various cancers, and its role in tumorigenesis is an area of active research. Studies suggest that COX-2-mediated lipid peroxidation, leading to the formation of reactive species like 4-oxo-2(E)-nonenal from 15(S)-hydroperoxyeicosatetraenoic acid (15(S)-HPETE), could contribute to DNA damage and potentially contribute to cancer development []. Additionally, the downregulation of 15-PGDH in certain cancers might lead to decreased production of anti-proliferative 15-oxo-ETE, further promoting tumor growth []. Therefore, understanding the complex interplay between COX-2, 15-PGDH, and their downstream metabolites like this compound, 11-oxo-ETE, and 15-oxo-ETE is crucial for developing targeted cancer therapies.
体外研究产品的免责声明和信息
请注意,BenchChem 上展示的所有文章和产品信息仅供信息参考。 BenchChem 上可购买的产品专为体外研究设计,这些研究在生物体外进行。体外研究,源自拉丁语 "in glass",涉及在受控实验室环境中使用细胞或组织进行的实验。重要的是要注意,这些产品没有被归类为药物或药品,他们没有得到 FDA 的批准,用于预防、治疗或治愈任何医疗状况、疾病或疾病。我们必须强调,将这些产品以任何形式引入人类或动物的身体都是法律严格禁止的。遵守这些指南对确保研究和实验的法律和道德标准的符合性至关重要。