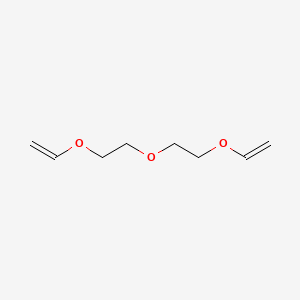
二甘醇二乙烯基醚
描述
Diethylene glycol divinyl ether is a useful research compound. Its molecular formula is C8H14O3 and its molecular weight is 158.19 g/mol. The purity is usually 95%.
The exact mass of the compound Diethylene glycol divinyl ether is unknown and the complexity rating of the compound is unknown. The compound has been submitted to the National Cancer Institute (NCI) for testing and evaluation and the Cancer Chemotherapy National Service Center (NSC) number is 6117. The United Nations designated GHS hazard class pictogram is Irritant, and the GHS signal word is WarningThe storage condition is unknown. Please store according to label instructions upon receipt of goods.
BenchChem offers high-quality Diethylene glycol divinyl ether suitable for many research applications. Different packaging options are available to accommodate customers' requirements. Please inquire for more information about Diethylene glycol divinyl ether including the price, delivery time, and more detailed information at info@benchchem.com.
科学研究应用
有机合成
二甘醇二乙烯基醚是有机合成中重要的原料和中间体 . 由于其双官能团性质,它可以用来合成各种有机化合物。
制药
在制药行业,二甘醇二乙烯基醚用作合成各种药物的中间体 . 它的独特化学性质使其适用于创建复杂的药物化合物。
农用化学品
二甘醇二乙烯基醚也用于农用化学品的生产 . 它可用于合成杀虫剂、除草剂和其他农业化学品。
染料
在染料行业,二甘醇二乙烯基醚用作原料 . 它可用于生产各种染料和颜料。
凝胶聚合物电解质
二甘醇二乙烯基醚可用于形成锂离子电池的凝胶聚合物电解质 . 这种应用在储能领域尤为重要。
光引发剂
二甘醇二乙烯基醚可用于通过乙烯基酯单体的阳离子聚合研究光引发剂 . 这是聚合物化学领域的重要应用。
作用机制
- DEGDVE is a colorless and transparent liquid with a boiling point of 198°C under atmospheric pressure . It is miscible with alcohol, ether, ester, and aromatic hydrocarbons but only slightly soluble in water.
- As a reactive diluent, DEGDVE is commonly used in UV- and peroxide-cured adhesives and sealants . Its presence modifies the curing process by affecting polymerization and cross-linking.
Mode of Action
生化分析
Biochemical Properties
It is known to be a reactive diluent, suggesting that it may interact with various enzymes, proteins, and other biomolecules
Cellular Effects
It is known to cause irritation of the digestive tract and respiratory tract, suggesting that it may have some impact on cell function
Molecular Mechanism
It is known to be a reactive diluent, suggesting that it may interact with biomolecules and potentially influence enzyme activity or gene expression
Temporal Effects in Laboratory Settings
It is known to be light-sensitive, suggesting that its effects may change over time
Dosage Effects in Animal Models
It is known to cause irritation, suggesting that it may have toxic or adverse effects at high doses
Metabolic Pathways
It is known to be metabolized in the liver by enzyme NAD-dependent alcohol dehydrogenase (ADH) to a hydrogen ion, NADH, and 2-hydroxyethoxyacetaldehyde (C4H8O3)
Transport and Distribution
It is known to be a liquid at room temperature, suggesting that it may be transported and distributed within cells and tissues
Subcellular Localization
It is known to be a liquid at room temperature, suggesting that it may be localized in various compartments or organelles within the cell
属性
IUPAC Name |
1-ethenoxy-2-(2-ethenoxyethoxy)ethane | |
---|---|---|
Source | PubChem | |
URL | https://pubchem.ncbi.nlm.nih.gov | |
Description | Data deposited in or computed by PubChem | |
InChI |
InChI=1S/C8H14O3/c1-3-9-5-7-11-8-6-10-4-2/h3-4H,1-2,5-8H2 | |
Source | PubChem | |
URL | https://pubchem.ncbi.nlm.nih.gov | |
Description | Data deposited in or computed by PubChem | |
InChI Key |
SAMJGBVVQUEMGC-UHFFFAOYSA-N | |
Source | PubChem | |
URL | https://pubchem.ncbi.nlm.nih.gov | |
Description | Data deposited in or computed by PubChem | |
Canonical SMILES |
C=COCCOCCOC=C | |
Source | PubChem | |
URL | https://pubchem.ncbi.nlm.nih.gov | |
Description | Data deposited in or computed by PubChem | |
Molecular Formula |
C8H14O3 | |
Source | PubChem | |
URL | https://pubchem.ncbi.nlm.nih.gov | |
Description | Data deposited in or computed by PubChem | |
Related CAS |
25215-94-5 | |
Record name | Diethylene glycol divinyl ether homopolymer | |
Source | CAS Common Chemistry | |
URL | https://commonchemistry.cas.org/detail?cas_rn=25215-94-5 | |
Description | CAS Common Chemistry is an open community resource for accessing chemical information. Nearly 500,000 chemical substances from CAS REGISTRY cover areas of community interest, including common and frequently regulated chemicals, and those relevant to high school and undergraduate chemistry classes. This chemical information, curated by our expert scientists, is provided in alignment with our mission as a division of the American Chemical Society. | |
Explanation | The data from CAS Common Chemistry is provided under a CC-BY-NC 4.0 license, unless otherwise stated. | |
DSSTOX Substance ID |
DTXSID6044985 | |
Record name | {2-[2-(Ethenyloxy)ethoxy]ethoxy}ethene | |
Source | EPA DSSTox | |
URL | https://comptox.epa.gov/dashboard/DTXSID6044985 | |
Description | DSSTox provides a high quality public chemistry resource for supporting improved predictive toxicology. | |
Molecular Weight |
158.19 g/mol | |
Source | PubChem | |
URL | https://pubchem.ncbi.nlm.nih.gov | |
Description | Data deposited in or computed by PubChem | |
Physical Description |
Liquid | |
Record name | Ethene, 1,1'-[oxybis(2,1-ethanediyloxy)]bis- | |
Source | EPA Chemicals under the TSCA | |
URL | https://www.epa.gov/chemicals-under-tsca | |
Description | EPA Chemicals under the Toxic Substances Control Act (TSCA) collection contains information on chemicals and their regulations under TSCA, including non-confidential content from the TSCA Chemical Substance Inventory and Chemical Data Reporting. | |
CAS No. |
764-99-8 | |
Record name | Diethylene glycol divinyl ether | |
Source | CAS Common Chemistry | |
URL | https://commonchemistry.cas.org/detail?cas_rn=764-99-8 | |
Description | CAS Common Chemistry is an open community resource for accessing chemical information. Nearly 500,000 chemical substances from CAS REGISTRY cover areas of community interest, including common and frequently regulated chemicals, and those relevant to high school and undergraduate chemistry classes. This chemical information, curated by our expert scientists, is provided in alignment with our mission as a division of the American Chemical Society. | |
Explanation | The data from CAS Common Chemistry is provided under a CC-BY-NC 4.0 license, unless otherwise stated. | |
Record name | Diethylene glycol divinyl ether | |
Source | ChemIDplus | |
URL | https://pubchem.ncbi.nlm.nih.gov/substance/?source=chemidplus&sourceid=0000764998 | |
Description | ChemIDplus is a free, web search system that provides access to the structure and nomenclature authority files used for the identification of chemical substances cited in National Library of Medicine (NLM) databases, including the TOXNET system. | |
Record name | Diethylene glycol divinyl ether | |
Source | DTP/NCI | |
URL | https://dtp.cancer.gov/dtpstandard/servlet/dwindex?searchtype=NSC&outputformat=html&searchlist=6117 | |
Description | The NCI Development Therapeutics Program (DTP) provides services and resources to the academic and private-sector research communities worldwide to facilitate the discovery and development of new cancer therapeutic agents. | |
Explanation | Unless otherwise indicated, all text within NCI products is free of copyright and may be reused without our permission. Credit the National Cancer Institute as the source. | |
Record name | Ethene, 1,1'-[oxybis(2,1-ethanediyloxy)]bis- | |
Source | EPA Chemicals under the TSCA | |
URL | https://www.epa.gov/chemicals-under-tsca | |
Description | EPA Chemicals under the Toxic Substances Control Act (TSCA) collection contains information on chemicals and their regulations under TSCA, including non-confidential content from the TSCA Chemical Substance Inventory and Chemical Data Reporting. | |
Record name | {2-[2-(Ethenyloxy)ethoxy]ethoxy}ethene | |
Source | EPA DSSTox | |
URL | https://comptox.epa.gov/dashboard/DTXSID6044985 | |
Description | DSSTox provides a high quality public chemistry resource for supporting improved predictive toxicology. | |
Record name | 1,1'-[oxybis(ethyleneoxy)]diethylene | |
Source | European Chemicals Agency (ECHA) | |
URL | https://echa.europa.eu/substance-information/-/substanceinfo/100.011.030 | |
Description | The European Chemicals Agency (ECHA) is an agency of the European Union which is the driving force among regulatory authorities in implementing the EU's groundbreaking chemicals legislation for the benefit of human health and the environment as well as for innovation and competitiveness. | |
Explanation | Use of the information, documents and data from the ECHA website is subject to the terms and conditions of this Legal Notice, and subject to other binding limitations provided for under applicable law, the information, documents and data made available on the ECHA website may be reproduced, distributed and/or used, totally or in part, for non-commercial purposes provided that ECHA is acknowledged as the source: "Source: European Chemicals Agency, http://echa.europa.eu/". Such acknowledgement must be included in each copy of the material. ECHA permits and encourages organisations and individuals to create links to the ECHA website under the following cumulative conditions: Links can only be made to webpages that provide a link to the Legal Notice page. | |
Record name | DIETHYLENE GLYCOL DIVINYL ETHER | |
Source | FDA Global Substance Registration System (GSRS) | |
URL | https://gsrs.ncats.nih.gov/ginas/app/beta/substances/ABM90Y2G95 | |
Description | The FDA Global Substance Registration System (GSRS) enables the efficient and accurate exchange of information on what substances are in regulated products. Instead of relying on names, which vary across regulatory domains, countries, and regions, the GSRS knowledge base makes it possible for substances to be defined by standardized, scientific descriptions. | |
Explanation | Unless otherwise noted, the contents of the FDA website (www.fda.gov), both text and graphics, are not copyrighted. They are in the public domain and may be republished, reprinted and otherwise used freely by anyone without the need to obtain permission from FDA. Credit to the U.S. Food and Drug Administration as the source is appreciated but not required. | |
Synthesis routes and methods
Procedure details
Retrosynthesis Analysis
AI-Powered Synthesis Planning: Our tool employs the Template_relevance Pistachio, Template_relevance Bkms_metabolic, Template_relevance Pistachio_ringbreaker, Template_relevance Reaxys, Template_relevance Reaxys_biocatalysis model, leveraging a vast database of chemical reactions to predict feasible synthetic routes.
One-Step Synthesis Focus: Specifically designed for one-step synthesis, it provides concise and direct routes for your target compounds, streamlining the synthesis process.
Accurate Predictions: Utilizing the extensive PISTACHIO, BKMS_METABOLIC, PISTACHIO_RINGBREAKER, REAXYS, REAXYS_BIOCATALYSIS database, our tool offers high-accuracy predictions, reflecting the latest in chemical research and data.
Strategy Settings
Precursor scoring | Relevance Heuristic |
---|---|
Min. plausibility | 0.01 |
Model | Template_relevance |
Template Set | Pistachio/Bkms_metabolic/Pistachio_ringbreaker/Reaxys/Reaxys_biocatalysis |
Top-N result to add to graph | 6 |
Feasible Synthetic Routes
Q1: What is the structural characterization of diethylene glycol divinyl ether?
A1: Diethylene glycol divinyl ether has the molecular formula C8H14O3 and a molecular weight of 158.19 g/mol. While specific spectroscopic data is not provided in the provided research papers, it can be characterized using techniques like 1H NMR and MALDI-TOF MS to analyze its structure and purity. [, ]
Q2: How is diethylene glycol divinyl ether used in polymer synthesis?
A2: Diethylene glycol divinyl ether is a valuable monomer in polymer chemistry. It can undergo cationic polymerization, where an initiator like hydrogen chloride/zinc chloride facilitates the opening of its vinyl groups, leading to polymer chain growth. [] The presence of oxygen atoms in its structure influences its polymerization behavior compared to divinyl ethers with oligomethylene chains. []
Q3: Can you elaborate on the influence of diethylene glycol divinyl ether's structure on its polymerization behavior?
A3: Research indicates that the oligooxyethylene spacer units in diethylene glycol divinyl ether influence its polymerization kinetics. Specifically, these units, when adjacent to unreacted vinyl groups, can interact with the positively charged active center during cationic polymerization. This interaction favors intramolecular crosslinking reactions, leading to the formation of soluble polymers even at high monomer conversions. []
Q4: What are the applications of diethylene glycol divinyl ether in creating functional materials?
A4: Diethylene glycol divinyl ether is used to create thermosensitive hydrogels. When copolymerized with monomers like ethylene glycol vinyl ether and butyl vinyl ether, it forms hydrogels that exhibit volume phase transition in response to temperature changes. [, ] These hydrogels have potential in applications like controlled drug release.
Q5: How is diethylene glycol divinyl ether used in drug delivery systems?
A5: Diethylene glycol divinyl ether-based thermosensitive hydrogels can be loaded with drugs like chlorhexidine for controlled release. [] The drug release rate can be tailored by adjusting the hydrogel composition and crosslinking density. The temperature responsiveness of these hydrogels allows for on-demand drug release at specific temperatures. []
Q6: How does diethylene glycol divinyl ether contribute to the performance of organic thin-film transistors (OTFTs)?
A7: Diethylene glycol divinyl ether is a component in polymer dielectrics used in OTFTs. For example, it is copolymerized with 2-cyanoethyl acrylate to form poly(2-cyanoethyl acrylate-co-diethylene glycol divinyl ether), or p(CEA-co-DEGDVE). While the cyanide functionality introduces polarity to the dielectric, which can hinder charge transport, using alkylated organic semiconductors can mitigate these negative effects, leading to improved OTFT performance. []
体外研究产品的免责声明和信息
请注意,BenchChem 上展示的所有文章和产品信息仅供信息参考。 BenchChem 上可购买的产品专为体外研究设计,这些研究在生物体外进行。体外研究,源自拉丁语 "in glass",涉及在受控实验室环境中使用细胞或组织进行的实验。重要的是要注意,这些产品没有被归类为药物或药品,他们没有得到 FDA 的批准,用于预防、治疗或治愈任何医疗状况、疾病或疾病。我们必须强调,将这些产品以任何形式引入人类或动物的身体都是法律严格禁止的。遵守这些指南对确保研究和实验的法律和道德标准的符合性至关重要。