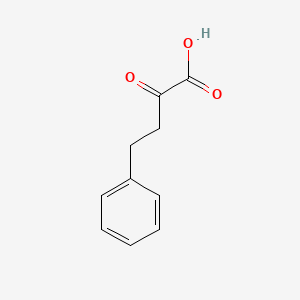
2-Oxo-4-phenylbutyric acid
描述
2-Oxo-4-phenylbutyric acid is a member of benzenes.
作用机制
Target of Action
The primary target of 2-oxo-4-phenylbutanoic acid (2-OPBA) is phenylalanine dehydrogenase (PheDH) . PheDH is an enzyme that plays a crucial role in the synthesis of the pharmaceutical chiral building block L-homophenylalanine (L-HPA) . This compound is used in the production of several blockbuster pharmaceuticals, such as angiotensin-converting enzyme inhibitors .
Mode of Action
The interaction of 2-OPBA with its target, PheDH, involves a process called direct reductive amination . In this process, 2-OPBA is catalyzed by PheDH, resulting in the synthesis of L-HPA . The sole expense of this process is ammonia, and water is the only byproduct .
Biochemical Pathways
The biochemical pathway affected by 2-OPBA involves the conversion of 2-OPBA to L-HPA, catalyzed by PheDH . This process is highly attractive in the synthesis of L-HPA, which serves as a versatile chiral building block for the synthesis of several pharmaceuticals .
Pharmacokinetics
It’s worth noting that the biocatalytic process involving 2-opba and phedh has been reported to have high catalytic efficiency .
Result of Action
The result of the action of 2-OPBA is the production of L-HPA . L-HPA is an important precursor for the production of angiotensin-converting enzyme inhibitors, which are significant drugs for preventing the formation of angiotensin II and lowering blood pressure .
Action Environment
The action of 2-OPBA is influenced by environmental factors such as substrate loading and the presence of indispensable cofactors . For instance, the viability of the biocatalytic process involving 2-OPBA and PheDH is markedly affected by insufficient substrate loading and high costs of the indispensable cofactors . A highly efficient and economic biocatalytic process for l-hpa has been established by coupling genetically modified phedh and formate dehydrogenase .
生化分析
Biochemical Properties
2-oxo-4-phenylbutanoic acid plays a crucial role in biochemical reactions, particularly in the synthesis of chiral building blocks such as L-homophenylalanine. This compound is involved in the direct reductive amination process catalyzed by phenylalanine dehydrogenase, where it is converted to L-homophenylalanine with the consumption of ammonia and the production of water as a byproduct . The interaction between 2-oxo-4-phenylbutanoic acid and phenylalanine dehydrogenase involves binding to the enzyme’s active site, where it undergoes a reduction reaction . Additionally, 2-oxo-4-phenylbutanoic acid can interact with other enzymes such as glucose dehydrogenase in paired reaction systems to enhance catalytic efficiency .
Cellular Effects
2-oxo-4-phenylbutanoic acid influences various cellular processes, including cell signaling pathways, gene expression, and cellular metabolism. In particular, the compound has been shown to affect the synthesis of L-homophenylalanine, an unnatural aromatic amino acid that serves as a chiral building block for pharmaceuticals . The presence of 2-oxo-4-phenylbutanoic acid in cells can lead to changes in gene expression related to amino acid metabolism and enzyme activity. Furthermore, the compound’s involvement in reductive amination reactions can impact cellular redox states and metabolic fluxes .
Molecular Mechanism
The molecular mechanism of 2-oxo-4-phenylbutanoic acid involves its interaction with specific enzymes, leading to enzyme activation or inhibition. For example, the compound binds to the active site of phenylalanine dehydrogenase, where it undergoes a reductive amination reaction to form L-homophenylalanine . This process involves the transfer of electrons from the enzyme’s cofactor to the keto group of 2-oxo-4-phenylbutanoic acid, resulting in the formation of an amino acid product . Additionally, the compound can interact with other enzymes such as glucose dehydrogenase, which can further modulate its biochemical activity .
Temporal Effects in Laboratory Settings
In laboratory settings, the effects of 2-oxo-4-phenylbutanoic acid can change over time due to factors such as stability, degradation, and long-term impacts on cellular function. The compound is relatively stable under standard storage conditions, but its activity can be influenced by factors such as temperature and pH . Over time, 2-oxo-4-phenylbutanoic acid may undergo degradation, leading to a decrease in its effectiveness in biochemical reactions . Long-term studies have shown that the compound can have sustained effects on cellular metabolism and enzyme activity, particularly in the context of amino acid synthesis .
Dosage Effects in Animal Models
The effects of 2-oxo-4-phenylbutanoic acid in animal models vary with different dosages. At low doses, the compound can effectively participate in biochemical reactions without causing adverse effects . At higher doses, 2-oxo-4-phenylbutanoic acid may exhibit toxic effects, including disruptions in metabolic processes and enzyme activity . Threshold effects have been observed, where the compound’s impact on cellular function becomes more pronounced at specific concentration levels . It is important to carefully control the dosage to avoid potential toxicity in animal studies .
Metabolic Pathways
2-oxo-4-phenylbutanoic acid is involved in several metabolic pathways, including the synthesis of L-homophenylalanine. The compound interacts with enzymes such as phenylalanine dehydrogenase and glucose dehydrogenase, which facilitate its conversion to amino acid products . These interactions can affect metabolic fluxes and the levels of various metabolites within cells . Additionally, 2-oxo-4-phenylbutanoic acid can participate in other biochemical pathways related to amino acid metabolism and redox reactions .
Transport and Distribution
Within cells and tissues, 2-oxo-4-phenylbutanoic acid is transported and distributed through interactions with specific transporters and binding proteins. The compound’s localization and accumulation can be influenced by factors such as its chemical properties and the presence of transport proteins . Studies have shown that 2-oxo-4-phenylbutanoic acid can be effectively transported across cellular membranes, allowing it to participate in intracellular biochemical reactions . The distribution of the compound within tissues can also impact its overall biochemical activity .
Subcellular Localization
The subcellular localization of 2-oxo-4-phenylbutanoic acid is an important factor in determining its activity and function. The compound can be directed to specific cellular compartments or organelles through targeting signals and post-translational modifications . For example, 2-oxo-4-phenylbutanoic acid may localize to the mitochondria, where it can participate in metabolic reactions related to energy production and redox balance . The compound’s localization can also influence its interactions with enzymes and other biomolecules, further modulating its biochemical effects .
属性
IUPAC Name |
2-oxo-4-phenylbutanoic acid | |
---|---|---|
Source | PubChem | |
URL | https://pubchem.ncbi.nlm.nih.gov | |
Description | Data deposited in or computed by PubChem | |
InChI |
InChI=1S/C10H10O3/c11-9(10(12)13)7-6-8-4-2-1-3-5-8/h1-5H,6-7H2,(H,12,13) | |
Source | PubChem | |
URL | https://pubchem.ncbi.nlm.nih.gov | |
Description | Data deposited in or computed by PubChem | |
InChI Key |
PPKAIMDMNWBOKN-UHFFFAOYSA-N | |
Source | PubChem | |
URL | https://pubchem.ncbi.nlm.nih.gov | |
Description | Data deposited in or computed by PubChem | |
Canonical SMILES |
C1=CC=C(C=C1)CCC(=O)C(=O)O | |
Source | PubChem | |
URL | https://pubchem.ncbi.nlm.nih.gov | |
Description | Data deposited in or computed by PubChem | |
Molecular Formula |
C10H10O3 | |
Source | PubChem | |
URL | https://pubchem.ncbi.nlm.nih.gov | |
Description | Data deposited in or computed by PubChem | |
DSSTOX Substance ID |
DTXSID10221224 | |
Record name | 2-Oxo-4-phenylbutyric acid | |
Source | EPA DSSTox | |
URL | https://comptox.epa.gov/dashboard/DTXSID10221224 | |
Description | DSSTox provides a high quality public chemistry resource for supporting improved predictive toxicology. | |
Molecular Weight |
178.18 g/mol | |
Source | PubChem | |
URL | https://pubchem.ncbi.nlm.nih.gov | |
Description | Data deposited in or computed by PubChem | |
CAS No. |
710-11-2 | |
Record name | α-Oxobenzenebutanoic acid | |
Source | CAS Common Chemistry | |
URL | https://commonchemistry.cas.org/detail?cas_rn=710-11-2 | |
Description | CAS Common Chemistry is an open community resource for accessing chemical information. Nearly 500,000 chemical substances from CAS REGISTRY cover areas of community interest, including common and frequently regulated chemicals, and those relevant to high school and undergraduate chemistry classes. This chemical information, curated by our expert scientists, is provided in alignment with our mission as a division of the American Chemical Society. | |
Explanation | The data from CAS Common Chemistry is provided under a CC-BY-NC 4.0 license, unless otherwise stated. | |
Record name | 2-Oxo-4-phenylbutyric acid | |
Source | ChemIDplus | |
URL | https://pubchem.ncbi.nlm.nih.gov/substance/?source=chemidplus&sourceid=0000710112 | |
Description | ChemIDplus is a free, web search system that provides access to the structure and nomenclature authority files used for the identification of chemical substances cited in National Library of Medicine (NLM) databases, including the TOXNET system. | |
Record name | 2-Oxo-4-phenylbutyric acid | |
Source | EPA DSSTox | |
URL | https://comptox.epa.gov/dashboard/DTXSID10221224 | |
Description | DSSTox provides a high quality public chemistry resource for supporting improved predictive toxicology. | |
Record name | 2-oxo-4-phenylbutyric acid | |
Source | European Chemicals Agency (ECHA) | |
URL | https://echa.europa.eu/substance-information/-/substanceinfo/100.010.834 | |
Description | The European Chemicals Agency (ECHA) is an agency of the European Union which is the driving force among regulatory authorities in implementing the EU's groundbreaking chemicals legislation for the benefit of human health and the environment as well as for innovation and competitiveness. | |
Explanation | Use of the information, documents and data from the ECHA website is subject to the terms and conditions of this Legal Notice, and subject to other binding limitations provided for under applicable law, the information, documents and data made available on the ECHA website may be reproduced, distributed and/or used, totally or in part, for non-commercial purposes provided that ECHA is acknowledged as the source: "Source: European Chemicals Agency, http://echa.europa.eu/". Such acknowledgement must be included in each copy of the material. ECHA permits and encourages organisations and individuals to create links to the ECHA website under the following cumulative conditions: Links can only be made to webpages that provide a link to the Legal Notice page. | |
Synthesis routes and methods I
Procedure details
Synthesis routes and methods II
Procedure details
Synthesis routes and methods III
Procedure details
Retrosynthesis Analysis
AI-Powered Synthesis Planning: Our tool employs the Template_relevance Pistachio, Template_relevance Bkms_metabolic, Template_relevance Pistachio_ringbreaker, Template_relevance Reaxys, Template_relevance Reaxys_biocatalysis model, leveraging a vast database of chemical reactions to predict feasible synthetic routes.
One-Step Synthesis Focus: Specifically designed for one-step synthesis, it provides concise and direct routes for your target compounds, streamlining the synthesis process.
Accurate Predictions: Utilizing the extensive PISTACHIO, BKMS_METABOLIC, PISTACHIO_RINGBREAKER, REAXYS, REAXYS_BIOCATALYSIS database, our tool offers high-accuracy predictions, reflecting the latest in chemical research and data.
Strategy Settings
Precursor scoring | Relevance Heuristic |
---|---|
Min. plausibility | 0.01 |
Model | Template_relevance |
Template Set | Pistachio/Bkms_metabolic/Pistachio_ringbreaker/Reaxys/Reaxys_biocatalysis |
Top-N result to add to graph | 6 |
Feasible Synthetic Routes
Q1: What is the molecular formula, weight, and relevant spectroscopic data for 2-Oxo-4-phenylbutyric acid?
A1: this compound has the molecular formula C10H10O3 and a molecular weight of 178.18 g/mol. While specific spectroscopic data wasn't detailed in the provided research, typical characterization would involve techniques like NMR (Nuclear Magnetic Resonance), IR (Infrared Spectroscopy), and Mass Spectrometry.
Q2: How is this compound used in the production of pharmaceuticals?
A2: OPBA serves as a prochiral intermediate in the synthesis of ACEI medications []. These medications are widely used for treating hypertension and congestive heart failure.
Q3: Can you elaborate on the enzymatic synthesis of L-Homophenylalanine (L-HPA) using this compound?
A3: L-HPA, a precursor for ACEIs, can be produced through the reductive amination of OPBA. This reaction is catalyzed by enzymes like L-phenylalanine dehydrogenase [] or meso-diaminopimelate dehydrogenase (DAPDH) [] and often employs a coupled enzyme system for cofactor regeneration [, , ].
Q4: Are there any alternative enzymatic pathways for L-HPA synthesis using OPBA?
A4: Yes, researchers have explored alternative pathways using enzymes like aspartate aminotransferase (AAT) [, , , ]. Engineered E. coli strains expressing modified AAT demonstrated efficient conversion of OPBA to L-HPA using L-lysine as an amino donor [, ].
Q5: What advantages do biocatalytic processes offer in the synthesis of L-HPA from OPBA?
A5: Biocatalytic processes, especially those employing engineered enzymes, offer high enantioselectivity, yielding L-HPA with high optical purity, a critical factor for pharmaceutical applications [, , , , , ].
Q6: How do structural modifications of enzymes involved in OPBA biotransformation affect their activity and selectivity?
A6: Research on enzymes like phenylalanine dehydrogenase (PheDH) highlights the importance of steric hindrance engineering []. Introducing specific mutations within the active site can significantly impact substrate binding and catalytic efficiency. For instance, the triple-site mutant V309G/L306V/V144G in Bacillus badius PheDH showed a remarkable 12.9-fold increase in catalytic efficiency (kcat/Km) compared to the wild-type enzyme [].
Q7: Are there any studies on engineering meso-diaminopimelate dehydrogenase (DAPDH) for improved activity towards OPBA?
A7: Yes, structure-guided mutagenesis of DAPDH from Symbiobacterium thermophilum led to the development of a double-site mutant, W121L/H227I. This mutant displayed significantly enhanced activity towards various 2-keto acids, including bulky substrates like OPBA []. This modification highlights the potential of protein engineering to expand the substrate scope and improve the catalytic efficiency of enzymes for the synthesis of valuable chiral compounds.
Q8: What strategies are employed to enhance the stability and reusability of enzymes used in OPBA biotransformation?
A8: Immobilization of enzymes, such as D-lactate dehydrogenase (D-nLDH) and formate dehydrogenase (FDH), onto solid supports like cotton cloth has been investigated to improve stability and facilitate continuous processes []. This approach allows for enzyme reuse and simplifies downstream processing.
Q9: How is this compound typically synthesized, and how is the reaction optimized?
A9: A common method involves the condensation of ethyl 3-phenylpropionate with diethyl oxalate, followed by hydrolysis []. Optimization often focuses on parameters like reactant ratios, reaction times, and temperature, as highlighted in studies aiming for high OPBA yields [, ].
Q10: Are there alternative routes or starting materials for the synthesis of compounds typically derived from this compound?
A10: While the provided research focuses primarily on OPBA as a key intermediate, exploring alternative synthetic routes for ACE inhibitors is an active area of research. Direct asymmetric hydrogenation of related compounds, like 2-oxo-4-arylbut-3-enoic acids, has shown promise in producing 2-hydroxy-4-arylbutanoic acids, which are also intermediates for ACE inhibitors [].
体外研究产品的免责声明和信息
请注意,BenchChem 上展示的所有文章和产品信息仅供信息参考。 BenchChem 上可购买的产品专为体外研究设计,这些研究在生物体外进行。体外研究,源自拉丁语 "in glass",涉及在受控实验室环境中使用细胞或组织进行的实验。重要的是要注意,这些产品没有被归类为药物或药品,他们没有得到 FDA 的批准,用于预防、治疗或治愈任何医疗状况、疾病或疾病。我们必须强调,将这些产品以任何形式引入人类或动物的身体都是法律严格禁止的。遵守这些指南对确保研究和实验的法律和道德标准的符合性至关重要。