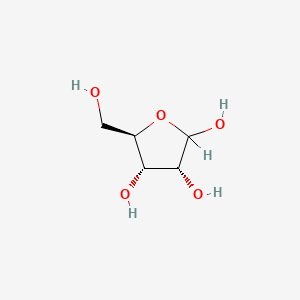
D-呋喃核糖
描述
D-ribofuranose is a five-membered ring sugar molecule that is a component of RNA and DNA. It is also a precursor to important coenzymes such as flavin mononucleotide (FMN) and flavin adenine dinucleotide (FAD). This molecule has been the subject of extensive scientific research due to its crucial role in biological processes.
科学研究应用
Chiral Recognition
D-ribofuranose plays a significant role in the chiral recognition of biological molecules . A simple and convenient approach for the chiral recognition of ribose has been developed using β-cyclodextrin (β-CD)-coated Ag NPs . This method is fast, convenient, low cost, and can be operated outside the laboratory .
Conformational Studies
D-ribofuranose and its derivatives have been synthesized and studied in terms of their conformational preferences . Based on the 1H NMR spectra, DFT calculations, and X-ray analysis, the E0-like and E4-like conformations adopted by these furanosides are identified .
Role in Ribonucleic Acid (RNA)
Ribose is the central molecular unit in ribose nucleic acid (RNA) . Ribonucleosides, which are representatives of this group of compounds, play essential functions in living organisms . They are involved in intermediary metabolism, cell signaling, and the biosynthesis of macromolecules .
Origin of Life Studies
Ribose is a key molecule in the study of many persistent scientific mysteries, such as the origin of life . Ribose and its sister pentoses can be made under alkaline conditions from simple organic precursors known to exist in interstellar space and presumably available on early Earth .
Medical Applications
Ribonucleosides and their derivatives are profoundly explored in medicine as anticancer, antibacterial, and antiviral agents . For example, Molnupiravir, a ribonucleoside with proven activity against a number of RNA viruses is currently tested against SARS-CoV-2 .
Role in Antibiotics
Some aminoglycoside-aminocyclitol antibiotics, namely ribostamycin, butirosin B, neomycin, and paromomycin contain an O-β-D-ribofuranoside ring in their structure . It has been suggested that the presence of the ribofuranose ring in these antibiotics may improve the bacterial ribosome selectivity .
作用机制
Target of Action
D-Ribofuranose, also known as ribofuranose, primarily targets several enzymes. These include Hypoxanthine-guanine phosphoribosyltransferase, Orotate phosphoribosyltransferase, Uridine-cytidine kinase-like 1, Adenine phosphoribosyltransferase, and Anthranilate phosphoribosyltransferase . These enzymes play crucial roles in various biochemical processes, including the biosynthesis of histidine, tryptophan, and purine and pyrimidine nucleotides .
Mode of Action
D-Ribofuranose interacts with its targets by participating in the transfer of the phosphoribosyl group of 5-phosphorylribose-1-pyrophosphate (PRPP) to anthranilate, yielding N-(5’-phosphoribosyl)-anthranilate (PRA) . This interaction is essential for the biosynthesis of histidine, tryptophan, and purine and pyrimidine nucleotides .
Biochemical Pathways
D-Ribofuranose is involved in several biochemical pathways. It plays a key role in the Pentose Phosphate Pathway, Pyrimidine Metabolism, and Purine Metabolism . These pathways are essential for the production of nucleotides and energy in the form of ATP.
Pharmacokinetics
It’s known that d-ribofuranose can be phosphorylated to form 5-phosphoribosyl 1-pyrophosphate (prpp), a key molecule in the biosynthesis of nucleotides .
Result of Action
The action of D-Ribofuranose results in the production of key substances for the biosynthesis of histidine, tryptophan, and purine and pyrimidine nucleotides . These nucleotides are essential for the synthesis of RNA and DNA, playing a crucial role in encoding, transmitting, and expressing genetic information in living organisms .
Action Environment
The action of D-Ribofuranose can be influenced by environmental factors such as temperature. For instance, a study showed that a steady temperature gradient may steer an open isomerisation network into a non-equilibrium steady state where furanose is boosted beyond the limits set by equilibrium thermodynamics . This suggests that environmental conditions can significantly impact the action and efficacy of D-Ribofuranose.
属性
IUPAC Name |
(3R,4S,5R)-5-(hydroxymethyl)oxolane-2,3,4-triol | |
---|---|---|
Source | PubChem | |
URL | https://pubchem.ncbi.nlm.nih.gov | |
Description | Data deposited in or computed by PubChem | |
InChI |
InChI=1S/C5H10O5/c6-1-2-3(7)4(8)5(9)10-2/h2-9H,1H2/t2-,3-,4-,5?/m1/s1 | |
Source | PubChem | |
URL | https://pubchem.ncbi.nlm.nih.gov | |
Description | Data deposited in or computed by PubChem | |
InChI Key |
HMFHBZSHGGEWLO-SOOFDHNKSA-N | |
Source | PubChem | |
URL | https://pubchem.ncbi.nlm.nih.gov | |
Description | Data deposited in or computed by PubChem | |
Canonical SMILES |
C(C1C(C(C(O1)O)O)O)O | |
Source | PubChem | |
URL | https://pubchem.ncbi.nlm.nih.gov | |
Description | Data deposited in or computed by PubChem | |
Isomeric SMILES |
C([C@@H]1[C@H]([C@H](C(O1)O)O)O)O | |
Source | PubChem | |
URL | https://pubchem.ncbi.nlm.nih.gov | |
Description | Data deposited in or computed by PubChem | |
Molecular Formula |
C5H10O5 | |
Source | PubChem | |
URL | https://pubchem.ncbi.nlm.nih.gov | |
Description | Data deposited in or computed by PubChem | |
DSSTOX Substance ID |
DTXSID201317333 | |
Record name | D-Ribofuranose | |
Source | EPA DSSTox | |
URL | https://comptox.epa.gov/dashboard/DTXSID201317333 | |
Description | DSSTox provides a high quality public chemistry resource for supporting improved predictive toxicology. | |
Molecular Weight |
150.13 g/mol | |
Source | PubChem | |
URL | https://pubchem.ncbi.nlm.nih.gov | |
Description | Data deposited in or computed by PubChem | |
Physical Description |
Solid | |
Record name | D-Ribose | |
Source | Human Metabolome Database (HMDB) | |
URL | http://www.hmdb.ca/metabolites/HMDB0000283 | |
Description | The Human Metabolome Database (HMDB) is a freely available electronic database containing detailed information about small molecule metabolites found in the human body. | |
Explanation | HMDB is offered to the public as a freely available resource. Use and re-distribution of the data, in whole or in part, for commercial purposes requires explicit permission of the authors and explicit acknowledgment of the source material (HMDB) and the original publication (see the HMDB citing page). We ask that users who download significant portions of the database cite the HMDB paper in any resulting publications. | |
CAS RN |
613-83-2 | |
Record name | D-Ribofuranose | |
Source | CAS Common Chemistry | |
URL | https://commonchemistry.cas.org/detail?cas_rn=613-83-2 | |
Description | CAS Common Chemistry is an open community resource for accessing chemical information. Nearly 500,000 chemical substances from CAS REGISTRY cover areas of community interest, including common and frequently regulated chemicals, and those relevant to high school and undergraduate chemistry classes. This chemical information, curated by our expert scientists, is provided in alignment with our mission as a division of the American Chemical Society. | |
Explanation | The data from CAS Common Chemistry is provided under a CC-BY-NC 4.0 license, unless otherwise stated. | |
Record name | D-Ribofuranose | |
Source | ChemIDplus | |
URL | https://pubchem.ncbi.nlm.nih.gov/substance/?source=chemidplus&sourceid=0000613832 | |
Description | ChemIDplus is a free, web search system that provides access to the structure and nomenclature authority files used for the identification of chemical substances cited in National Library of Medicine (NLM) databases, including the TOXNET system. | |
Record name | D-Ribofuranose | |
Source | EPA DSSTox | |
URL | https://comptox.epa.gov/dashboard/DTXSID201317333 | |
Description | DSSTox provides a high quality public chemistry resource for supporting improved predictive toxicology. | |
Record name | D-Ribose | |
Source | Human Metabolome Database (HMDB) | |
URL | http://www.hmdb.ca/metabolites/HMDB0000283 | |
Description | The Human Metabolome Database (HMDB) is a freely available electronic database containing detailed information about small molecule metabolites found in the human body. | |
Explanation | HMDB is offered to the public as a freely available resource. Use and re-distribution of the data, in whole or in part, for commercial purposes requires explicit permission of the authors and explicit acknowledgment of the source material (HMDB) and the original publication (see the HMDB citing page). We ask that users who download significant portions of the database cite the HMDB paper in any resulting publications. | |
Melting Point |
95 °C | |
Record name | D-Ribose | |
Source | Human Metabolome Database (HMDB) | |
URL | http://www.hmdb.ca/metabolites/HMDB0000283 | |
Description | The Human Metabolome Database (HMDB) is a freely available electronic database containing detailed information about small molecule metabolites found in the human body. | |
Explanation | HMDB is offered to the public as a freely available resource. Use and re-distribution of the data, in whole or in part, for commercial purposes requires explicit permission of the authors and explicit acknowledgment of the source material (HMDB) and the original publication (see the HMDB citing page). We ask that users who download significant portions of the database cite the HMDB paper in any resulting publications. | |
Retrosynthesis Analysis
AI-Powered Synthesis Planning: Our tool employs the Template_relevance Pistachio, Template_relevance Bkms_metabolic, Template_relevance Pistachio_ringbreaker, Template_relevance Reaxys, Template_relevance Reaxys_biocatalysis model, leveraging a vast database of chemical reactions to predict feasible synthetic routes.
One-Step Synthesis Focus: Specifically designed for one-step synthesis, it provides concise and direct routes for your target compounds, streamlining the synthesis process.
Accurate Predictions: Utilizing the extensive PISTACHIO, BKMS_METABOLIC, PISTACHIO_RINGBREAKER, REAXYS, REAXYS_BIOCATALYSIS database, our tool offers high-accuracy predictions, reflecting the latest in chemical research and data.
Strategy Settings
Precursor scoring | Relevance Heuristic |
---|---|
Min. plausibility | 0.01 |
Model | Template_relevance |
Template Set | Pistachio/Bkms_metabolic/Pistachio_ringbreaker/Reaxys/Reaxys_biocatalysis |
Top-N result to add to graph | 6 |
Feasible Synthetic Routes
Q & A
Q1: What is the molecular formula and weight of D-ribofuranose?
A1: D-ribofuranose has the molecular formula C5H10O5 and a molecular weight of 150.13 g/mol.
Q2: What spectroscopic data is available to characterize D-ribofuranose?
A2: Various spectroscopic techniques are employed to characterize D-ribofuranose, including:
- Nuclear Magnetic Resonance (NMR) Spectroscopy: NMR provides valuable insights into the structure and conformation of ribofuranose, particularly the arrangement of atoms and the orientation of the hydroxyl groups. [, , ]
- Infrared (IR) Spectroscopy: IR spectroscopy helps identify functional groups present in ribofuranose, such as the hydroxyl and ether groups. [, , ]
- Mass Spectrometry (MS): MS helps determine the molecular weight and fragmentation patterns of ribofuranose and its derivatives. [, ]
Q3: What is the significance of the circular hydrogen bond networks on the surface of beta-ribofuranose in aqueous solution?
A3: Ab initio molecular dynamics simulations have revealed that the circular hydrogen bond networks, involving two ribofuranose oxygens and three water molecules, are influenced by the orientation of the hydroxymethyl group. These networks, found near the ring oxygen, exhibit both homodromic and antidromic arrangements, highlighting the role of electronic properties and polarization effects in their formation. []
Q4: How does D-ribofuranose perform under various conditions?
A4: D-ribofuranose exhibits stability under a range of conditions, but its reactivity can be influenced by factors like pH, temperature, and the presence of specific reagents.
Q5: Are there any specific material compatibility considerations for D-ribofuranose?
A5: The compatibility of D-ribofuranose with other materials depends on the specific application and reaction conditions. Researchers should consider potential side reactions or degradation products.
Q6: What are some key intermediates and synthetic routes for preparing D-ribofuranose derivatives?
A6: Several synthetic routes have been developed for D-ribofuranose derivatives:
- Starting from D-ribose: This approach often involves protection and deprotection steps to selectively modify the hydroxyl groups. Common protecting groups include acetyl, benzoyl, and isopropylidene groups. [, , , , ]
- Utilizing D-ribonolactone derivatives: Lactone derivatives, like D-ribonic-γ-lactone, provide another starting point for synthesizing modified ribofuranose derivatives. These reactions may involve Grignard reagents or other organometallic compounds. [, ]
- Employing enzymatic methods: Enzymatic hydrolysis offers a regioselective approach for deacetylating ribofuranose derivatives, allowing access to specific isomers and facilitating further modifications. []
Q7: What are some examples of modified ribofuranose derivatives and their applications?
A7: Modified ribofuranose derivatives have diverse applications:
- Fluorinated derivatives: Fluorinated ribofuranose analogues, like 1′-deoxy-1′-(2,4,6-trifluorophenyl)-ß-D-ribofuranose and 1′-deoxy-1′-(2,4,5-trifluorophenyl)-ß-D-ribofuranose, have been synthesized and incorporated into RNA oligonucleotides for structural investigations. These modifications allow for studying base stacking, base pairing, and hydrogen bonding in RNA. [, ]
- C-aryl glycosides: The synthesis of C-aryl glycosides, like 1-deoxy-1-phenyl-β-D-ribofuranose, has allowed for incorporating hydrophobic base analogs into RNA. These modifications help study the effects of hydrophobicity on RNA structure and function, particularly in the context of hammerhead ribozymes. []
- Branched ribofuranose derivatives: Nucleosides containing branched ribose structures, like 2′-C-difluoromethylribonucleosides, show promise as therapeutic agents. These compounds can be incorporated into oligonucleotides using enzymatic methods, potentially leading to modified RNA with altered stability and biological activity. []
Q8: How do structural modifications of D-ribofuranose influence its biological activity?
A8: Modifications to the ribofuranose structure can significantly impact the biological activity of its derivatives:
- Base modifications: Replacing the natural nucleobases in ribonucleosides with modified analogs can significantly alter binding affinity to target proteins and influence biological activity. For example, replacing adenine in adenophostin A with imidazole or purine affects its activity as an agonist for 1D-myo-inositol 1,4,5-trisphosphate receptors. []
- Sugar modifications: Changes to the sugar moiety, like fluorination or the introduction of bulky substituents, can affect the conformation, stability, and interactions of ribofuranose derivatives with enzymes or receptors. [, , ]
Q9: Have any specific biological activities been reported for D-ribofuranose derivatives?
A9: Research has identified several biological activities associated with D-ribofuranose derivatives:
- Antiviral activity: Some modified ribofuranose derivatives, particularly those containing sulfated acetamido groups, have shown promising antiviral activity against HIV. [] Additionally, quinolone ribonucleosides with modifications at the C-3 position have been synthesized and are under investigation for their potential antiviral activity against HSV-1 and HIV-1. []
- Anticancer activity: The synthesis of 1,2,3-tri-O-acetyl-5-deoxy-D-ribofuranose, a crucial intermediate for the anticancer drug Capecitabine, highlights the potential of modified ribofuranose derivatives in developing chemotherapeutic agents. [, ]
- Anti-inflammatory and analgesic activities: Derivatives of 3-O-benzyl-4-C-(hydroxymethyl)-1,2-O-isopropylidene-α-D-ribofuranose have demonstrated promising analgesic and anti-inflammatory effects in animal models. These findings suggest potential applications for modified ribofuranose derivatives in treating pain and inflammation. []
Q10: What is the significance of d-ribofuranose in the context of the T1 side chain of Salmonella lipopolysaccharide?
A10: Isotope labeling studies have provided insights into the biosynthesis of d-ribofuranose residues in the T1 side chains of Salmonella lipopolysaccharide. These studies suggest that the d-ribofuranose residues are likely synthesized through conventional metabolic pathways involving the hexose monophosphate shunt and transketolase-transaldolase reactions, rather than through a direct conversion from aldohexoses. []
Q11: How is computational chemistry being used to study D-ribofuranose and its derivatives?
A11: Computational chemistry plays a vital role in understanding the properties and behavior of D-ribofuranose:
体外研究产品的免责声明和信息
请注意,BenchChem 上展示的所有文章和产品信息仅供信息参考。 BenchChem 上可购买的产品专为体外研究设计,这些研究在生物体外进行。体外研究,源自拉丁语 "in glass",涉及在受控实验室环境中使用细胞或组织进行的实验。重要的是要注意,这些产品没有被归类为药物或药品,他们没有得到 FDA 的批准,用于预防、治疗或治愈任何医疗状况、疾病或疾病。我们必须强调,将这些产品以任何形式引入人类或动物的身体都是法律严格禁止的。遵守这些指南对确保研究和实验的法律和道德标准的符合性至关重要。