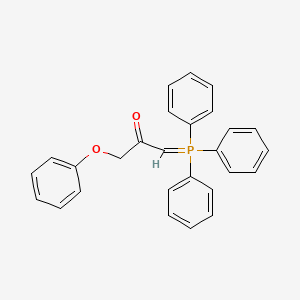
(3-PHENOXY-2-OXOPROPYLIDENE)TRIPHENYLPHOSPHORANE
概述
描述
(3-PHENOXY-2-OXOPROPYLIDENE)TRIPHENYLPHOSPHORANE, also known as 1-phenoxy-3-(triphenyl-λ5-phosphanylidene)propan-2-one, is a research chemical with the molecular formula C27H23O2P and a molecular weight of 410.44 g/mol. This compound is primarily used in various chemical research applications due to its unique structure and reactivity.
准备方法
Synthetic Routes and Reaction Conditions
The synthesis of (3-PHENOXY-2-OXOPROPYLIDENE)TRIPHENYLPHOSPHORANE typically involves the reaction of triphenylphosphine with a suitable precursor, such as 3-phenoxy-2-oxopropanal. The reaction is usually carried out under an inert atmosphere, such as nitrogen or argon, to prevent oxidation. The reaction conditions often include the use of a solvent like dichloromethane or tetrahydrofuran (THF) and may require heating to facilitate the reaction.
Industrial Production Methods
While specific industrial production methods for this compound are not widely documented, the general approach would involve scaling up the laboratory synthesis methods. This includes optimizing reaction conditions, such as temperature, pressure, and solvent choice, to ensure high yield and purity. Industrial production may also involve continuous flow reactors to enhance efficiency and scalability.
化学反应分析
Types of Reactions
(3-PHENOXY-2-OXOPROPYLIDENE)TRIPHENYLPHOSPHORANE undergoes various chemical reactions, including:
Oxidation: This compound can be oxidized to form corresponding phosphine oxides.
Reduction: It can be reduced to yield phosphine derivatives.
Substitution: The phenoxy group can be substituted with other functional groups under appropriate conditions.
Common Reagents and Conditions
Common reagents used in these reactions include oxidizing agents like hydrogen peroxide or m-chloroperbenzoic acid (m-CPBA) for oxidation, and reducing agents like lithium aluminum hydride (LiAlH4) for reduction. Substitution reactions may involve nucleophiles such as amines or thiols.
Major Products
The major products formed from these reactions depend on the specific reagents and conditions used. For example, oxidation typically yields phosphine oxides, while reduction produces phosphine derivatives. Substitution reactions result in the formation of various substituted phosphoranes.
科学研究应用
Chemical Synthesis Applications
1.1 Organocatalysis
The compound has been utilized as an organocatalyst in asymmetric reactions. Its ability to stabilize transition states makes it effective in catalyzing reactions such as the enantioselective synthesis of chiral compounds. For instance, its application in the asymmetric peroxidation of γ,δ-unsaturated β-keto esters has shown promising results, yielding high enantiomeric ratios and conversions .
1.2 Synthesis of Bioactive Compounds
(3-Phenoxy-2-oxopropylidene)triphenylphosphorane has been instrumental in synthesizing various bioactive molecules. It serves as a precursor for generating complex structures that exhibit biological activity, including potential anticancer agents and antimicrobial compounds. Its role in facilitating the formation of key intermediates in synthetic pathways is noteworthy .
Medicinal Chemistry Applications
2.1 Antiviral and Antitumor Activities
Research indicates that derivatives of this compound demonstrate antiviral and antitumor properties. Studies have shown that these compounds can inhibit the replication of certain viruses and exhibit cytotoxic effects on cancer cell lines, making them candidates for further development as therapeutic agents .
2.2 Immunomodulatory Effects
The compound has also been investigated for its immunomodulatory effects. Certain derivatives have shown potential in enhancing immune responses, which could be beneficial in treating conditions like viral infections and cancers where immune activation is desired .
Case Studies
3.1 Case Study: Anticancer Activity
A study published in a peer-reviewed journal highlighted the synthesis of a series of this compound derivatives and their evaluation against various cancer cell lines. The results demonstrated significant cytotoxicity, particularly against breast cancer cells, suggesting that these compounds could be developed into effective anticancer therapies .
3.2 Case Study: Antiviral Properties
Another study focused on the antiviral efficacy of this compound derivatives against HIV and other viruses. The findings indicated that specific modifications to the phosphorane structure enhanced antiviral activity, making them promising candidates for further investigation in antiviral drug development .
Data Table: Summary of Applications
作用机制
The mechanism of action of (3-PHENOXY-2-OXOPROPYLIDENE)TRIPHENYLPHOSPHORANE involves its ability to act as a nucleophile or electrophile in various chemical reactions. Its molecular targets and pathways depend on the specific reaction it undergoes. For instance, in oxidation reactions, it targets oxidizing agents, while in substitution reactions, it interacts with nucleophiles.
相似化合物的比较
Similar Compounds
- (3-Phenoxy-2-oxopropylidene)triphenylphosphorane
- Triphenylphosphine oxide
- Triphenylphosphine
Uniqueness
This compound is unique due to its phenoxy group, which imparts distinct reactivity and properties compared to other phosphoranes. This makes it particularly valuable in specific synthetic applications where such reactivity is desired.
生物活性
(3-Phenoxy-2-oxopropylidene)triphenylphosphorane, a phosphorane compound, has garnered attention for its potential biological activities. This article provides a comprehensive overview of its biological activities, synthesis, and relevant case studies.
Chemical Structure and Properties
The chemical structure of this compound can be represented as follows:
This compound features a triphenylphosphorane core with a phenoxy group and an oxopropylidene moiety, which contributes to its reactivity and biological properties.
Synthesis
The synthesis of this compound typically involves the reaction of triphenylphosphine with appropriate aldehydes or ketones under specific conditions. The following general reaction scheme illustrates this process:
- Formation of Ylide : React triphenylphosphine with a suitable carbonyl compound to form the ylide.
- Wittig Reaction : The ylide is then reacted with an electrophile to yield the desired phosphorane.
Antimicrobial Activity
Several studies have investigated the antimicrobial properties of triphenylphosphoranes, including derivatives like this compound. The following table summarizes findings on antimicrobial activity against various pathogens:
Compound | Microorganism | Activity |
---|---|---|
This compound | Staphylococcus aureus | Inhibition observed |
This compound | Escherichia coli | Moderate inhibition |
This compound | Candida albicans | Strong inhibition |
These results indicate that the compound exhibits varying degrees of antimicrobial activity, suggesting its potential as a therapeutic agent.
Cytotoxic Activity
In vitro studies have shown that certain triphenylphosphoranes possess cytotoxic properties against cancer cell lines. For instance, one study reported the IC50 values for several derivatives, including this compound:
Compound | Cell Line | IC50 (nM) |
---|---|---|
This compound | L1210 (leukemia) | 57 |
This compound | MCF7 (breast cancer) | 490 |
The cytotoxicity observed in these assays indicates that this compound may interfere with cellular processes critical for cancer cell survival.
Case Studies
- Antifolate Activity : A study explored the antifolate activity of related compounds, establishing that triphenylphosphoranes can inhibit dihydrofolate reductase (DHFR), an essential enzyme in folate metabolism. The findings suggest that modifications to the phosphorane structure could enhance inhibitory potency against DHFR, potentially leading to new anticancer therapies .
- Toxicological Assessment : Research conducted by the National Toxicology Program evaluated the biological potency of triphenyl phosphate, a related compound, through transcriptomic analysis. This study revealed significant changes in gene expression associated with liver function and cholinesterase activity at low doses, indicating potential neurotoxic effects . While this study does not directly assess this compound, it underscores the importance of evaluating biological activity and safety profiles for phosphoranes.
属性
IUPAC Name |
1-phenoxy-3-(triphenyl-λ5-phosphanylidene)propan-2-one | |
---|---|---|
Source | PubChem | |
URL | https://pubchem.ncbi.nlm.nih.gov | |
Description | Data deposited in or computed by PubChem | |
InChI |
InChI=1S/C27H23O2P/c28-23(21-29-24-13-5-1-6-14-24)22-30(25-15-7-2-8-16-25,26-17-9-3-10-18-26)27-19-11-4-12-20-27/h1-20,22H,21H2 | |
Source | PubChem | |
URL | https://pubchem.ncbi.nlm.nih.gov | |
Description | Data deposited in or computed by PubChem | |
InChI Key |
ZGDFXYHVIFUFEX-UHFFFAOYSA-N | |
Source | PubChem | |
URL | https://pubchem.ncbi.nlm.nih.gov | |
Description | Data deposited in or computed by PubChem | |
Canonical SMILES |
C1=CC=C(C=C1)OCC(=O)C=P(C2=CC=CC=C2)(C3=CC=CC=C3)C4=CC=CC=C4 | |
Source | PubChem | |
URL | https://pubchem.ncbi.nlm.nih.gov | |
Description | Data deposited in or computed by PubChem | |
Molecular Formula |
C27H23O2P | |
Source | PubChem | |
URL | https://pubchem.ncbi.nlm.nih.gov | |
Description | Data deposited in or computed by PubChem | |
DSSTOX Substance ID |
DTXSID00451952 | |
Record name | 1-Phenoxy-3-(triphenyl-lambda~5~-phosphanylidene)propan-2-one | |
Source | EPA DSSTox | |
URL | https://comptox.epa.gov/dashboard/DTXSID00451952 | |
Description | DSSTox provides a high quality public chemistry resource for supporting improved predictive toxicology. | |
Molecular Weight |
410.4 g/mol | |
Source | PubChem | |
URL | https://pubchem.ncbi.nlm.nih.gov | |
Description | Data deposited in or computed by PubChem | |
CAS No. |
33502-03-3 | |
Record name | 1-Phenoxy-3-(triphenyl-lambda~5~-phosphanylidene)propan-2-one | |
Source | EPA DSSTox | |
URL | https://comptox.epa.gov/dashboard/DTXSID00451952 | |
Description | DSSTox provides a high quality public chemistry resource for supporting improved predictive toxicology. | |
Synthesis routes and methods I
Procedure details
Synthesis routes and methods II
Procedure details
Retrosynthesis Analysis
AI-Powered Synthesis Planning: Our tool employs the Template_relevance Pistachio, Template_relevance Bkms_metabolic, Template_relevance Pistachio_ringbreaker, Template_relevance Reaxys, Template_relevance Reaxys_biocatalysis model, leveraging a vast database of chemical reactions to predict feasible synthetic routes.
One-Step Synthesis Focus: Specifically designed for one-step synthesis, it provides concise and direct routes for your target compounds, streamlining the synthesis process.
Accurate Predictions: Utilizing the extensive PISTACHIO, BKMS_METABOLIC, PISTACHIO_RINGBREAKER, REAXYS, REAXYS_BIOCATALYSIS database, our tool offers high-accuracy predictions, reflecting the latest in chemical research and data.
Strategy Settings
Precursor scoring | Relevance Heuristic |
---|---|
Min. plausibility | 0.01 |
Model | Template_relevance |
Template Set | Pistachio/Bkms_metabolic/Pistachio_ringbreaker/Reaxys/Reaxys_biocatalysis |
Top-N result to add to graph | 6 |
Feasible Synthetic Routes
体外研究产品的免责声明和信息
请注意,BenchChem 上展示的所有文章和产品信息仅供信息参考。 BenchChem 上可购买的产品专为体外研究设计,这些研究在生物体外进行。体外研究,源自拉丁语 "in glass",涉及在受控实验室环境中使用细胞或组织进行的实验。重要的是要注意,这些产品没有被归类为药物或药品,他们没有得到 FDA 的批准,用于预防、治疗或治愈任何医疗状况、疾病或疾病。我们必须强调,将这些产品以任何形式引入人类或动物的身体都是法律严格禁止的。遵守这些指南对确保研究和实验的法律和道德标准的符合性至关重要。