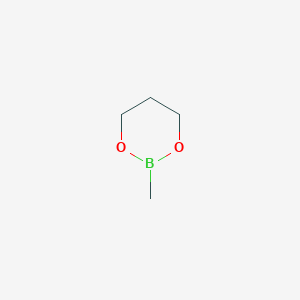
2-Methyl-1,3,2-dioxaborinane
描述
2-Methyl-1,3,2-dioxaborinane is a cyclic boron ester with the molecular formula C4H9BO2 It is a member of the dioxaborinane family, which are known for their unique structural properties and reactivity
准备方法
Synthetic Routes and Reaction Conditions
2-Methyl-1,3,2-dioxaborinane can be synthesized through the reaction of boric acid with 1,3-propanediol in the presence of a methylating agent. The reaction typically involves heating the reactants under reflux conditions to facilitate the formation of the cyclic ester. The process can be represented by the following equation:
B(OH)3+HOCH2CH2CH2OH→C4H9BO2+3H2O
Industrial Production Methods
Industrial production of this compound follows similar synthetic routes but on a larger scale. The process involves the use of continuous flow reactors to ensure efficient mixing and heat transfer. The reaction conditions are optimized to maximize yield and purity, often involving the use of catalysts to enhance the reaction rate.
化学反应分析
Types of Reactions
2-Methyl-1,3,2-dioxaborinane undergoes various chemical reactions, including:
Oxidation: The compound can be oxidized to form boronic acids or borates.
Reduction: Reduction reactions can convert it into borohydrides.
Substitution: It can participate in nucleophilic substitution reactions, where the boron atom is replaced by other nucleophiles.
Common Reagents and Conditions
Oxidation: Common oxidizing agents include hydrogen peroxide and peracids.
Reduction: Reducing agents such as lithium aluminum hydride are often used.
Substitution: Nucleophiles like amines and alcohols can react with this compound under mild conditions.
Major Products
Oxidation: Boronic acids and borates.
Reduction: Borohydrides.
Substitution: Various substituted boron compounds depending on the nucleophile used.
科学研究应用
2-Methyl-1,3,2-dioxaborinane has several applications in scientific research:
Organic Synthesis: It is used as a building block for the synthesis of complex organic molecules.
Material Science: The compound is utilized in the development of boron-containing polymers and materials with unique properties.
Biology and Medicine: Research is ongoing into its potential use in drug delivery systems and as a boron source in boron neutron capture therapy (BNCT) for cancer treatment.
Industry: It finds applications in the production of boron-based catalysts and as a reagent in various chemical processes.
作用机制
The mechanism of action of 2-Methyl-1,3,2-dioxaborinane involves its ability to form stable complexes with various molecules. The boron atom in the compound acts as a Lewis acid, accepting electron pairs from donor molecules. This property is exploited in catalysis and in the formation of stable adducts with other compounds. The molecular targets and pathways involved depend on the specific application and the nature of the interacting molecules.
相似化合物的比较
2-Methyl-1,3,2-dioxaborinane can be compared with other cyclic boron esters such as:
1,3,2-Dioxaborinane: Lacks the methyl group, resulting in different reactivity and stability.
2,2’-Bi-1,3,2-dioxaborinane: Contains two dioxaborinane units, offering unique properties for polymerization and material science applications.
2-Isobutyl-5-methyl-4-phenyl-1,3,2-dioxaborinane:
The uniqueness of this compound lies in its specific structural features and reactivity, making it a valuable compound in various fields of research and industry.
属性
IUPAC Name |
2-methyl-1,3,2-dioxaborinane | |
---|---|---|
Source | PubChem | |
URL | https://pubchem.ncbi.nlm.nih.gov | |
Description | Data deposited in or computed by PubChem | |
InChI |
InChI=1S/C4H9BO2/c1-5-6-3-2-4-7-5/h2-4H2,1H3 | |
Source | PubChem | |
URL | https://pubchem.ncbi.nlm.nih.gov | |
Description | Data deposited in or computed by PubChem | |
InChI Key |
KQVWFBVPBAQTES-UHFFFAOYSA-N | |
Source | PubChem | |
URL | https://pubchem.ncbi.nlm.nih.gov | |
Description | Data deposited in or computed by PubChem | |
Canonical SMILES |
B1(OCCCO1)C | |
Source | PubChem | |
URL | https://pubchem.ncbi.nlm.nih.gov | |
Description | Data deposited in or computed by PubChem | |
Molecular Formula |
C4H9BO2 | |
Source | PubChem | |
URL | https://pubchem.ncbi.nlm.nih.gov | |
Description | Data deposited in or computed by PubChem | |
DSSTOX Substance ID |
DTXSID30455645 | |
Record name | 2-methyl-1,3,2-dioxaborinane | |
Source | EPA DSSTox | |
URL | https://comptox.epa.gov/dashboard/DTXSID30455645 | |
Description | DSSTox provides a high quality public chemistry resource for supporting improved predictive toxicology. | |
Molecular Weight |
99.93 g/mol | |
Source | PubChem | |
URL | https://pubchem.ncbi.nlm.nih.gov | |
Description | Data deposited in or computed by PubChem | |
CAS No. |
51901-48-5 | |
Record name | 2-methyl-1,3,2-dioxaborinane | |
Source | EPA DSSTox | |
URL | https://comptox.epa.gov/dashboard/DTXSID30455645 | |
Description | DSSTox provides a high quality public chemistry resource for supporting improved predictive toxicology. | |
Retrosynthesis Analysis
AI-Powered Synthesis Planning: Our tool employs the Template_relevance Pistachio, Template_relevance Bkms_metabolic, Template_relevance Pistachio_ringbreaker, Template_relevance Reaxys, Template_relevance Reaxys_biocatalysis model, leveraging a vast database of chemical reactions to predict feasible synthetic routes.
One-Step Synthesis Focus: Specifically designed for one-step synthesis, it provides concise and direct routes for your target compounds, streamlining the synthesis process.
Accurate Predictions: Utilizing the extensive PISTACHIO, BKMS_METABOLIC, PISTACHIO_RINGBREAKER, REAXYS, REAXYS_BIOCATALYSIS database, our tool offers high-accuracy predictions, reflecting the latest in chemical research and data.
Strategy Settings
Precursor scoring | Relevance Heuristic |
---|---|
Min. plausibility | 0.01 |
Model | Template_relevance |
Template Set | Pistachio/Bkms_metabolic/Pistachio_ringbreaker/Reaxys/Reaxys_biocatalysis |
Top-N result to add to graph | 6 |
Feasible Synthetic Routes
体外研究产品的免责声明和信息
请注意,BenchChem 上展示的所有文章和产品信息仅供信息参考。 BenchChem 上可购买的产品专为体外研究设计,这些研究在生物体外进行。体外研究,源自拉丁语 "in glass",涉及在受控实验室环境中使用细胞或组织进行的实验。重要的是要注意,这些产品没有被归类为药物或药品,他们没有得到 FDA 的批准,用于预防、治疗或治愈任何医疗状况、疾病或疾病。我们必须强调,将这些产品以任何形式引入人类或动物的身体都是法律严格禁止的。遵守这些指南对确保研究和实验的法律和道德标准的符合性至关重要。