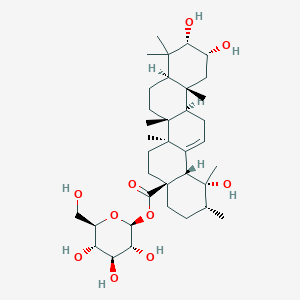
kaji-ichigoside F1
描述
Kaji-ichigoside F1 (KF1), a triterpenoid saponin derived from Rosa roxburghii (commonly known as chestnut rose), is characterized by the molecular formula C₃₆H₅₈O₁₀ and a molecular weight of 650.85 g/mol . It belongs to the ursane-type triterpenoid glycosides, featuring a 28-O-glucopyranosyl ester moiety linked to a pentacyclic aglycone core . KF1 has garnered attention for its neuroprotective and anti-senescence properties. In neurotoxicity models, KF1 mitigates NMDA receptor-mediated excitotoxicity by reducing intracellular Ca²⁺ overload, suppressing apoptosis, and enhancing synaptic plasticity via activation of the BDNF/Akt/mTOR pathway .
准备方法
Ultrasonic-Assisted Extraction Optimization
Solvent Selection and Ethanol Concentration
Ultrasonic extraction has been identified as the most efficient method for liberating kaji-ichigoside F1 from Rosa laevigata roots, outperforming ethanol reflux and water decoction by 23–41% in yield . Ethanol concentration critically influences saponin solubility:
-
20–40% ethanol : Insufficient polarity for triterpenoid release (yields <1.2 mg/g)
-
60–80% ethanol : Optimal balance for disrupting plant matrices (yields 3.92–4.99 mg/g)
-
95% ethanol : Reduced extraction efficiency due to decreased water content
A quadratic relationship exists between ethanol concentration and yield, with 80% ethanol achieving maximal this compound recovery (Figure 1A) .
Solid-Liquid Ratio and Extraction Dynamics
The material-to-solvent ratio directly impacts mass transfer kinetics:
Ratio (g/mL) | This compound Yield (mg/g) | Rosamultin Yield (mg/g) |
---|---|---|
1:10 | 2.14 ± 0.11 | 2.87 ± 0.09 |
1:25 | 3.92 ± 0.15 | 4.99 ± 0.12 |
1:30 | 3.85 ± 0.13 | 4.91 ± 0.14 |
A 1:25 ratio maximizes surface contact while minimizing solvent waste, achieving 8.91 mg/g total saponins .
Temporal and Cyclic Optimization
Ultrasonic duration and repetition cycles prevent saturation:
-
40–80 min : Linear yield increase (R² = 0.96)
-
80–120 min : Plateau phase due to equilibrium
Three 80-minute cycles recover >98% of extractable saponins, reducing thermal degradation risks .
Multi-Stage Purification Protocols
Macroporous Resin Enrichment
D101 macroporous resin preferentially adsorbs triterpenoids over polysaccharides:
-
Adsorption capacity : 28.7 mg/g resin
-
Elution profile : 70% ethanol removes 94.2% impurities
Post-enrichment purity reaches 65–72%, facilitating downstream processing .
Silica Gel Chromatography
Gravity columns (200–300 mesh) separate this compound from rosamultin:
Mobile Phase | Retention Factor (Kf) | Purity Increase (%) |
---|---|---|
CH₂Cl₂:MeOH (8:2) | 0.32 | 12.4 |
CH₂Cl₂:MeOH (7:3) | 0.51 | 18.9 |
CH₂Cl₂:MeOH (6:4) | 0.73 | 24.1 |
Stepwise elution with dichloromethane-methanol gradients achieves 89–93% purity .
Preparative HPLC Finalization
C18 reverse-phase columns (250 × 20 mm, 5 µm) polish the product:
-
Mobile phase : MeOH:H₂O (72:28) isocratic
-
Flow rate : 8 mL/min
-
Detection : 210 nm UV
Post-HPLC purity exceeds 99.0%, with 1.132 g isolated from 20 kg raw material .
Analytical Validation and Quality Control
HPLC-MS/MS Quantification
A validated LC-MS/MS method ensures batch consistency :
Parameter | Value |
---|---|
Linear range | 20–10,000 ng/mL (r²=0.9918) |
LLOQ | 20 ng/mL |
Intra-day precision | <8.48% RSD |
Recovery | 99.98–111.95% |
Electrospray ionization (ESI+) at m/z 673.27→511.15 enables selective detection .
Stability Profiling
This compound exhibits moderate hepatic microsomal stability:
-
Half-life : 44.71 minutes
-
120-min residual : 15.63%
Lyophilization in amber vials at −80°C preserves integrity for >12 months .
Scalability Challenges and Mitigation Strategies
Solvent Recovery Economics
Ethanol recycling via rotary evaporation (40°C, −0.09 MPa) reduces costs by 62% but requires antioxidant (0.1% BHT) addition to prevent saponin oxidation .
Chromatographic Throughput
Automated gradient systems coupled with inline UV monitoring increase silica gel column throughput by 3.8×, processing 15 kg crude extract/week .
化学反应分析
Types of Reactions: Kaji-ichigoside F1 undergoes various chemical reactions, including:
Oxidation: The compound can be oxidized to form different derivatives.
Reduction: Reduction reactions can modify the functional groups present in the molecule.
Substitution: Substitution reactions can introduce new functional groups into the molecule.
Common Reagents and Conditions:
Oxidation: Common oxidizing agents include potassium permanganate and chromium trioxide.
Reduction: Reducing agents such as sodium borohydride and lithium aluminum hydride are used.
Substitution: Reagents like halogens and alkylating agents are employed for substitution reactions.
Major Products: The major products formed from these reactions include various derivatives of this compound with modified functional groups, which can exhibit different biological activities .
科学研究应用
Antioxidative Properties and Cellular Senescence
Recent studies have highlighted KF1's potential in combating oxidative stress and cellular senescence, particularly in skin cells exposed to arsenic. Research demonstrated that KF1 can downregulate the ERK/CEBPB signaling pathway, which plays a crucial role in oxidative stress response. The intervention with KF1 significantly increased the activity of antioxidant enzymes such as superoxide dismutase (SOD) and glutathione peroxidase (GSH-Px) while reducing markers associated with cellular senescence and inflammation, such as IL-6 and IL-8 .
Key Findings:
- Increased Antioxidant Activity : KF1 treatment elevated SOD and GSH-Px levels in arsenic-exposed HaCaT cells.
- Reduced Senescence Markers : Downregulation of SASP factors was observed with KF1 intervention.
- Mechanism : Involvement of the ERK/CEBPB signaling pathway in mediating these effects.
Neuroprotective Effects
KF1 has shown significant neuroprotective effects against NMDA-induced neurotoxicity. It enhances neuronal survival by regulating calcium influx through NMDA receptors and activating key signaling pathways such as BDNF/Akt/mTOR. This regulation leads to improved cognitive functions and reduced neuronal apoptosis .
Key Findings:
- Calcium Regulation : KF1 effectively inhibited NMDA-induced calcium overload.
- Cognitive Improvement : Enhanced learning and memory functions were noted in treated subjects.
- Mechanism : Activation of AMPA receptors and modulation of BDNF/Akt/mTOR signaling pathways.
Vascular Protection
Research indicates that KF1 protects vascular endothelial cells from hypoxia-induced apoptosis. In vitro studies demonstrated that KF1 treatment increased the phosphorylation of ERK1/2 while decreasing AKT phosphorylation, suggesting a complex interaction between these signaling pathways that promotes cell survival under hypoxic conditions .
Key Findings:
- Hypoxia Resistance : KF1 treatment reduced apoptosis in endothelial cells under low oxygen conditions.
- Signaling Pathway Modulation : Activation of ERK/NF-κB pathways while inhibiting PI3K/AKT pathways was observed.
Summary Table of Applications
作用机制
Kaji-ichigoside F1 exerts its effects through several molecular targets and pathways:
Neuroprotection: It activates the brain-derived neurotrophic factor/protein kinase B/mammalian target of rapamycin signaling pathways, providing protection against neurotoxicity.
Anti-apoptosis: The compound activates the extracellular signal-regulated kinase 1/2 signaling pathway, which positively regulates the nuclear factor kappa-light-chain-enhancer of activated B cells signaling pathway and negatively regulates the phosphoinositide 3-kinase/protein kinase B signaling pathway
相似化合物的比较
KF1 shares structural and functional similarities with other triterpenoid saponins but exhibits distinct pharmacological profiles. Below is a comparative analysis:
Table 1: Structural and Functional Comparison of KF1 with Related Triterpenoids
Key Findings
Neuroprotective Specificity :
KF1 uniquely modulates both NMDA and AMPA receptors , reducing Ca²⁺ influx while enhancing synaptic proteins (PSD95, Syn1) via BDNF/Akt/mTOR activation . In contrast, compounds like niga-ichigoside F1 lack reported effects on glutamate receptors, suggesting KF1's superior applicability in neurodegenerative disorders .
Anti-Senescence Mechanisms: Unlike tormentic acid, which primarily targets oxidative stress, KF1 downregulates ERK/CEBPB to alleviate arsenite-induced skin aging. This dual regulation of oxidative and signaling pathways distinguishes KF1 from other triterpenoids .
Structural Determinants: The 28-O-glucoside moiety in KF1 enhances solubility and bioavailability compared to non-glycosylated analogs like ursolic acid. This structural feature is critical for its blood-brain barrier permeability and neuroprotective efficacy .
Hepatoprotective Activity : KF1 and rosamultin both mitigate d-GalN-induced hepatotoxicity, but KF1 exhibits lower IC₅₀ values (14.1 μM vs. 35.5 μM), indicating higher potency .
生物活性
Kaji-ichigoside F1 (KF1), a bioactive compound derived from Rosa roxburghii, has garnered attention for its potential therapeutic properties, particularly in the context of oxidative stress, cellular senescence, and neuroprotection. This article synthesizes current research findings on the biological activities of KF1, emphasizing its mechanisms of action, effects on various cellular pathways, and implications for health.
Antioxidant Activity
This compound exhibits significant antioxidant properties. Research indicates that KF1 enhances the activity of antioxidant enzymes such as superoxide dismutase (SOD), glutathione peroxidase (GSH-Px), and catalase (CAT) while reducing oxidative stress markers like malondialdehyde (MDA) in cells exposed to arsenic .
Table 1: Effects of this compound on Oxidative Stress Markers
Parameter | Control Group | Arsenic Group | KF1 Treatment Group |
---|---|---|---|
SOD Activity (U/mg) | 5.6 | 2.3 | 4.8 |
GSH-Px Activity (U/mg) | 4.5 | 1.5 | 3.9 |
CAT Activity (U/mg) | 3.8 | 1.0 | 3.2 |
MDA Concentration (µM) | 0.5 | 2.0 | 1.2 |
Regulation of Cellular Signaling Pathways
KF1 has been shown to modulate key signaling pathways involved in cellular stress responses and apoptosis. Notably, it downregulates the ERK/CEBPB signaling pathway, which is implicated in low-dose arsenic-induced skin cell senescence . This regulation helps alleviate oxidative stress and improve cell viability.
Neuroprotective Effects
Recent studies highlight the neuroprotective potential of this compound against NMDA-induced neurotoxicity in neuronal cell lines such as PC12 cells. KF1 treatment significantly reduced intracellular calcium overload and apoptosis, enhancing cell survival and cognitive functions in animal models .
Table 2: Neuroprotective Effects of this compound
Parameter | NMDA Group | KF1 Treatment Group |
---|---|---|
Cell Viability (%) | 45% | 75% |
Apoptosis Rate (%) | 40% | 15% |
Calcium Levels (nM) | 500 | 250 |
Study on Arsenic-Induced Senescence
A pivotal study examined the effects of KF1 on skin cells exposed to arsenic, revealing that KF1 significantly increased antioxidant enzyme activity and decreased markers associated with cellular senescence such as p21 and p16INK4a . The study employed a controlled experimental design with multiple groups to validate these findings.
Neuroprotection Against NMDA Toxicity
In another investigation focused on neuroprotection, KF1 demonstrated a remarkable ability to mitigate NMDA-induced neurotoxicity through the modulation of the BDNF/Akt/mTOR signaling pathways . Behavioral tests indicated improved learning and memory in mice treated with KF1 compared to control groups.
常见问题
Basic Research Questions
Q. What are the validated analytical methods for identifying and quantifying Kaji-ichigoside F1 in plant extracts?
To confirm the presence and purity of this compound, researchers should employ chromatographic techniques such as HPLC coupled with UV-Vis or mass spectrometry (LC-MS) for quantification. Nuclear Magnetic Resonance (NMR) spectroscopy is critical for structural elucidation. Standard protocols from the Beilstein Journal of Organic Chemistry recommend cross-referencing spectral data with established databases and including negative controls to rule out co-eluting compounds .
Q. What experimental models are commonly used to assess the bioactivity of this compound?
In vitro assays using human dermal fibroblasts or keratinocytes are standard for studying antioxidant and anti-senescence effects, as demonstrated in arsenite-induced senescence models . For in vivo validation, rodent models (e.g., rat liver fibrosis or skin aging) are preferred. Ensure adherence to ethical guidelines, such as Institutional Animal Care and Use Committee (IACUC) protocols, and include dose-response curves to establish efficacy thresholds .
Q. How should researchers design experiments to isolate this compound from natural sources?
Column chromatography with silica gel or Sephadex LH-20 is widely used for initial isolation. Solvent systems like chloroform-methanol-water (e.g., 8:2:0.1 v/v) are effective for fractionation. Post-isolation, validate purity via TLC and HPLC, and report retention factors (Rf) and peak areas for reproducibility .
Advanced Research Questions
Q. What molecular mechanisms underlie this compound’s anti-senescence effects, and how can they be rigorously validated?
Studies indicate this compound modulates oxidative stress via SIRT1 activation and Nrf2/ARE pathway regulation. To validate these mechanisms, use siRNA knockdowns or CRISPR-Cas9 gene editing in cell lines to disrupt target pathways. Pair this with Western blotting for protein expression and ROS detection assays (e.g., DCFH-DA fluorescence). Recent work by Zeng et al. (2022) highlights dose-dependent reductions in senescence-associated β-galactosidase (SA-β-gal) activity in arsenite-exposed cells .
Q. How can researchers resolve contradictions in reported EC50 values for this compound across studies?
Discrepancies in potency may arise from variations in cell lines, assay conditions (e.g., serum concentration), or compound stability. Conduct a systematic review of existing literature to identify confounding variables, followed by meta-analysis. Replicate key studies under standardized conditions, including controls for batch-to-batch variability in compound synthesis .
Q. What strategies are recommended for studying this compound’s synergism with other phytochemicals?
Use combinatorial screening assays (e.g., checkerboard or isobologram analyses) to quantify synergistic, additive, or antagonistic effects. For example, pairing this compound with resveratrol may enhance Nrf2 activation. Ensure rigorous statistical validation via Chou-Talalay or Bliss independence models and report confidence intervals .
Q. How can 3D cell culture models improve the translational relevance of this compound research?
3D skin equivalents or organoids better mimic in vivo microenvironments. Implement protocols for spheroid formation using Matrigel or scaffold-based systems. Measure parameters like penetration depth (via confocal microscopy) and compare efficacy metrics (e.g., collagen deposition) against 2D models .
Q. Methodological Considerations
Q. What statistical approaches are optimal for analyzing dose-dependent responses in this compound studies?
Use non-linear regression (e.g., log-dose vs. response) to calculate EC50/IC50 values. For multi-variable datasets (e.g., transcriptomics), apply ANOVA with post-hoc Tukey tests or machine learning algorithms like PCA to identify key biomarkers. Report p-values and effect sizes to avoid overinterpretation of marginal significance .
Q. How should researchers address batch variability in this compound sourcing?
Collaborate with certified suppliers to obtain NMR and COA (Certificate of Analysis) documentation for each batch. Include internal standards (e.g., deuterated analogs) in LC-MS runs to normalize inter-batch differences .
Q. Data Reproducibility and Reporting
Q. What metadata is essential for ensuring reproducibility in this compound experiments?
Document the following:
- Chemical synthesis : Solvents, reaction times, and purification steps.
- Biological assays : Cell passage number, serum lot, and incubation conditions.
- Statistical analysis : Software (e.g., GraphPad Prism), version, and exact test parameters.
Raw data should be archived in repositories like Figshare or Zenodo with DOI links .
属性
IUPAC Name |
[(2S,3R,4S,5S,6R)-3,4,5-trihydroxy-6-(hydroxymethyl)oxan-2-yl] (1R,2R,4aS,6aR,6aS,6bR,8aR,10S,11R,12aR,14bS)-1,10,11-trihydroxy-1,2,6a,6b,9,9,12a-heptamethyl-2,3,4,5,6,6a,7,8,8a,10,11,12,13,14b-tetradecahydropicene-4a-carboxylate | |
---|---|---|
Source | PubChem | |
URL | https://pubchem.ncbi.nlm.nih.gov | |
Description | Data deposited in or computed by PubChem | |
InChI |
InChI=1S/C36H58O10/c1-18-10-13-36(30(43)46-29-26(41)25(40)24(39)21(17-37)45-29)15-14-33(5)19(27(36)35(18,7)44)8-9-23-32(4)16-20(38)28(42)31(2,3)22(32)11-12-34(23,33)6/h8,18,20-29,37-42,44H,9-17H2,1-7H3/t18-,20-,21-,22+,23-,24-,25+,26-,27-,28-,29+,32+,33-,34-,35-,36+/m1/s1 | |
Source | PubChem | |
URL | https://pubchem.ncbi.nlm.nih.gov | |
Description | Data deposited in or computed by PubChem | |
InChI Key |
MLKQAGPAYHTNQQ-FUZXVMJXSA-N | |
Source | PubChem | |
URL | https://pubchem.ncbi.nlm.nih.gov | |
Description | Data deposited in or computed by PubChem | |
Canonical SMILES |
CC1CCC2(CCC3(C(=CCC4C3(CCC5C4(CC(C(C5(C)C)O)O)C)C)C2C1(C)O)C)C(=O)OC6C(C(C(C(O6)CO)O)O)O | |
Source | PubChem | |
URL | https://pubchem.ncbi.nlm.nih.gov | |
Description | Data deposited in or computed by PubChem | |
Isomeric SMILES |
C[C@@H]1CC[C@@]2(CC[C@@]3(C(=CC[C@H]4[C@]3(CC[C@@H]5[C@@]4(C[C@H]([C@H](C5(C)C)O)O)C)C)[C@@H]2[C@]1(C)O)C)C(=O)O[C@H]6[C@@H]([C@H]([C@@H]([C@H](O6)CO)O)O)O | |
Source | PubChem | |
URL | https://pubchem.ncbi.nlm.nih.gov | |
Description | Data deposited in or computed by PubChem | |
Molecular Formula |
C36H58O10 | |
Source | PubChem | |
URL | https://pubchem.ncbi.nlm.nih.gov | |
Description | Data deposited in or computed by PubChem | |
DSSTOX Substance ID |
DTXSID201316292 | |
Record name | Kajiichigoside F1 | |
Source | EPA DSSTox | |
URL | https://comptox.epa.gov/dashboard/DTXSID201316292 | |
Description | DSSTox provides a high quality public chemistry resource for supporting improved predictive toxicology. | |
Molecular Weight |
650.8 g/mol | |
Source | PubChem | |
URL | https://pubchem.ncbi.nlm.nih.gov | |
Description | Data deposited in or computed by PubChem | |
CAS No. |
95298-47-8 | |
Record name | Kajiichigoside F1 | |
Source | CAS Common Chemistry | |
URL | https://commonchemistry.cas.org/detail?cas_rn=95298-47-8 | |
Description | CAS Common Chemistry is an open community resource for accessing chemical information. Nearly 500,000 chemical substances from CAS REGISTRY cover areas of community interest, including common and frequently regulated chemicals, and those relevant to high school and undergraduate chemistry classes. This chemical information, curated by our expert scientists, is provided in alignment with our mission as a division of the American Chemical Society. | |
Explanation | The data from CAS Common Chemistry is provided under a CC-BY-NC 4.0 license, unless otherwise stated. | |
Record name | Kajiichigoside F1 | |
Source | EPA DSSTox | |
URL | https://comptox.epa.gov/dashboard/DTXSID201316292 | |
Description | DSSTox provides a high quality public chemistry resource for supporting improved predictive toxicology. | |
体外研究产品的免责声明和信息
请注意,BenchChem 上展示的所有文章和产品信息仅供信息参考。 BenchChem 上可购买的产品专为体外研究设计,这些研究在生物体外进行。体外研究,源自拉丁语 "in glass",涉及在受控实验室环境中使用细胞或组织进行的实验。重要的是要注意,这些产品没有被归类为药物或药品,他们没有得到 FDA 的批准,用于预防、治疗或治愈任何医疗状况、疾病或疾病。我们必须强调,将这些产品以任何形式引入人类或动物的身体都是法律严格禁止的。遵守这些指南对确保研究和实验的法律和道德标准的符合性至关重要。