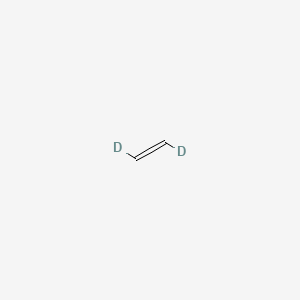
(Z)-Ethylene-1,2-d2
概述
描述
(Z)-Ethylene-1,2-d2 is a useful research compound. Its molecular formula is C2H4 and its molecular weight is 30.07 g/mol. The purity is usually 95%.
BenchChem offers high-quality this compound suitable for many research applications. Different packaging options are available to accommodate customers' requirements. Please inquire for more information about this compound including the price, delivery time, and more detailed information at info@benchchem.com.
生物活性
(Z)-Ethylene-1,2-d2, a deuterated analogue of ethylene, has garnered attention in various fields of research, particularly in understanding its biological activity and implications in biochemical processes. This article explores the compound's biological effects, mechanisms of action, and relevant case studies.
Overview of this compound
This compound is a deuterated version of ethylene where two hydrogen atoms are replaced by deuterium. This modification alters its physical properties and can influence its interaction with biological systems. Ethylene itself is a plant hormone that plays crucial roles in growth regulation, stress responses, and developmental processes.
- Gene Expression Modulation : Ethylene is known to regulate gene expression related to stress responses and growth cessation. Studies have shown that ethylene influences the expression of genes associated with cell wall modification and hypoxia tolerance in plants. For example, the application of ethylene can lead to the downregulation of genes involved in mitosis and DNA replication under stress conditions .
- Stress Response : Research indicates that this compound enhances tolerance to hypoxic conditions by modulating root growth and promoting the expression of hypoxia-responsive genes. This is critical for plant survival during flooding or waterlogging events .
- Reactive Oxygen Species (ROS) Homeostasis : Ethylene plays a role in managing oxidative stress by regulating the expression of peroxidases and other antioxidant enzymes. This regulation helps mitigate damage caused by ROS during environmental stress .
Case Studies
- Root Hypoxia Tolerance : A study demonstrated that ethylene pretreatment resulted in significant changes in gene expression profiles that enhance root hypoxia tolerance. Key genes associated with oxidative stress response were upregulated, indicating a protective mechanism against hypoxic damage .
- Latex Metabolism in Rubber Trees : Another investigation into rubber tree physiology revealed that ethylene application could activate latex metabolism under specific tapping conditions. This suggests that this compound may also influence metabolic pathways related to latex production .
Table 1: Summary of Biological Effects of this compound
Research Findings
Recent findings have emphasized the importance of ethylene in various biological processes beyond its traditional roles as a plant hormone. The use of deuterated compounds like this compound allows for more precise investigations into metabolic pathways due to their distinct isotopic signatures.
Implications for Future Research
The unique properties of this compound present opportunities for further exploration into:
- Plant Stress Physiology : Understanding how ethylene mediates stress responses can inform agricultural practices aimed at enhancing crop resilience.
- Metabolic Engineering : Insights gained from studying deuterated analogues can aid in developing crops with improved metabolic profiles for bioengineering applications.
科学研究应用
Spectroscopic Studies
Rotational Spectroscopy
(Z)-Ethylene-1,2-d2 is utilized in rotational spectroscopy to study molecular structures and dynamics. Its isotopic substitution allows for clearer spectral lines due to reduced vibrational coupling compared to its non-deuterated counterpart. For instance, a study identified (Z)-1,2-ethenediol (the enol form of glycolaldehyde) using high-resolution rotational spectroscopy. This research demonstrated the feasibility of detecting this compound in interstellar environments, enhancing our understanding of prebiotic chemistry and the origins of life on Earth .
Quantum-Chemical Calculations
The spectral parameters derived from quantum-chemical calculations align closely with experimental data for this compound. This alignment facilitates the identification of similar compounds in astrophysical contexts, supporting research into the chemical processes that may have led to life on Earth .
Catalysis
Silver-Catalyzed Reactions
this compound plays a crucial role in silver-catalyzed ethylene epoxidation processes. The presence of deuterium alters reaction kinetics and mechanisms, providing insights into the structure-sensitivity of catalysts used in producing ethylene oxide (EO). Studies indicate that variations in particle size and composition of silver catalysts significantly influence the efficiency of these reactions .
Applications in Polymer Chemistry
In polymer synthesis, this compound is employed to produce deuterated polyethylenes for use in advanced materials. These materials exhibit distinct properties that can be tailored for specific applications in packaging and other industries .
Biochemical Applications
Plant Hormone Studies
Ethylene is a vital plant hormone involved in regulating growth and responses to stress conditions such as hypoxia. Research indicates that deuterated forms like this compound can be used to trace metabolic pathways and understand ethylene's role in plant physiology under various environmental conditions. The incorporation of deuterium allows for precise tracking using mass spectrometry techniques .
属性
IUPAC Name |
1,2-dideuterioethene | |
---|---|---|
Source | PubChem | |
URL | https://pubchem.ncbi.nlm.nih.gov | |
Description | Data deposited in or computed by PubChem | |
InChI |
InChI=1S/C2H4/c1-2/h1-2H2/i1D,2D | |
Source | PubChem | |
URL | https://pubchem.ncbi.nlm.nih.gov | |
Description | Data deposited in or computed by PubChem | |
InChI Key |
VGGSQFUCUMXWEO-QDNHWIQGSA-N | |
Source | PubChem | |
URL | https://pubchem.ncbi.nlm.nih.gov | |
Description | Data deposited in or computed by PubChem | |
Canonical SMILES |
C=C | |
Source | PubChem | |
URL | https://pubchem.ncbi.nlm.nih.gov | |
Description | Data deposited in or computed by PubChem | |
Isomeric SMILES |
[2H]C=C[2H] | |
Source | PubChem | |
URL | https://pubchem.ncbi.nlm.nih.gov | |
Description | Data deposited in or computed by PubChem | |
Molecular Formula |
C2H4 | |
Source | PubChem | |
URL | https://pubchem.ncbi.nlm.nih.gov | |
Description | Data deposited in or computed by PubChem | |
Molecular Weight |
30.07 g/mol | |
Source | PubChem | |
URL | https://pubchem.ncbi.nlm.nih.gov | |
Description | Data deposited in or computed by PubChem | |
CAS No. |
2813-62-9 | |
Record name | (Z)-Ethylene-1,2-d2 | |
Source | ChemIDplus | |
URL | https://pubchem.ncbi.nlm.nih.gov/substance/?source=chemidplus&sourceid=0002813629 | |
Description | ChemIDplus is a free, web search system that provides access to the structure and nomenclature authority files used for the identification of chemical substances cited in National Library of Medicine (NLM) databases, including the TOXNET system. | |
Synthesis routes and methods I
Procedure details
Synthesis routes and methods II
Procedure details
Synthesis routes and methods III
Procedure details
Synthesis routes and methods IV
Procedure details
Retrosynthesis Analysis
AI-Powered Synthesis Planning: Our tool employs the Template_relevance Pistachio, Template_relevance Bkms_metabolic, Template_relevance Pistachio_ringbreaker, Template_relevance Reaxys, Template_relevance Reaxys_biocatalysis model, leveraging a vast database of chemical reactions to predict feasible synthetic routes.
One-Step Synthesis Focus: Specifically designed for one-step synthesis, it provides concise and direct routes for your target compounds, streamlining the synthesis process.
Accurate Predictions: Utilizing the extensive PISTACHIO, BKMS_METABOLIC, PISTACHIO_RINGBREAKER, REAXYS, REAXYS_BIOCATALYSIS database, our tool offers high-accuracy predictions, reflecting the latest in chemical research and data.
Strategy Settings
Precursor scoring | Relevance Heuristic |
---|---|
Min. plausibility | 0.01 |
Model | Template_relevance |
Template Set | Pistachio/Bkms_metabolic/Pistachio_ringbreaker/Reaxys/Reaxys_biocatalysis |
Top-N result to add to graph | 6 |
Feasible Synthetic Routes
体外研究产品的免责声明和信息
请注意,BenchChem 上展示的所有文章和产品信息仅供信息参考。 BenchChem 上可购买的产品专为体外研究设计,这些研究在生物体外进行。体外研究,源自拉丁语 "in glass",涉及在受控实验室环境中使用细胞或组织进行的实验。重要的是要注意,这些产品没有被归类为药物或药品,他们没有得到 FDA 的批准,用于预防、治疗或治愈任何医疗状况、疾病或疾病。我们必须强调,将这些产品以任何形式引入人类或动物的身体都是法律严格禁止的。遵守这些指南对确保研究和实验的法律和道德标准的符合性至关重要。