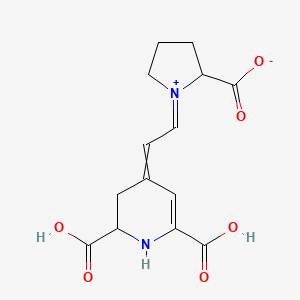
Indicaxanthin
概述
描述
Definition and Classification
Indicaxanthin (C14H16N2O6) is a betaxanthin, a subclass of betalain pigments found in plants[“]. Betalains are water-soluble nitrogen-containing pigments that replace anthocyanins in most families of the plant order Caryophyllales[“]. This compound is classified as a yellow-orange pigment, distinguishing it from the red-violet betacyanins[“].
Table 1: Classification of Betalain Pigments
Class | Subclass | Examples |
Betalains | Betacyanins | Betanin, lsobetanin |
Betaxanthins | This compound,Vulgaxanthin |
Sources
This compound is predominantly found in the following sources:
Cactus pear (Opuntia ficus-indica): The primary and most studied source[“].
Beets (Beta vulgaris): Contains this compound along with other betalains[“].
Mirabilis jalapa flowers: Another natural source of this pigment[“].
Red dragonfruit (Hylocereus costaricensis): Contains this compound among other betalains[“].
Case Study: Opuntia ficus-indica as a Source of this compound
A study by Gómez-Maqueo et al. analyzed the bioaccessibility of this compound from Opuntia ficus-indica fruit extracts. They reported bioaccessibility values of 58 ± 5% for the Colorada (yellow-orangish) variety[“]. This high bioaccessibility contributes to the potential health benefits of consuming cactus pear fruits.
Chemical Structure and Properties
This compound has a unique chemical structure that contributes to its biological activities and physicochemical properties.
Structure: this compound features a dihydropyridine ring system with two carboxylic acid groups and a vinyl group connecting to a pyrrolidine ring. This structure contributes to its amphiphilic nature, allowing interactions with both hydrophilic and lipophilic environments.
生物化学性质
Molecular Composition
Indicaxanthin (this compound) is a betaxanthin with a molecular weight of 308.29 g/mol3. Its structure features a dihydropyridine ring system connected to a pyrrolidine ring via a vinyl group, with two carboxylic acid groups[“]. This unique composition contributes to its amphiphilic nature, allowing interactions with both hydrophilic and lipophilic environments.
Table 1: Molecular Properties of this compound
Property | Value |
Molecular Formula | C14H16N206 |
Molecular Weight | 308.29 g/mol |
CAS Number | 2181-75-1 |
The molecule possesses ionizable carboxyl groups and a positive charge near the N1 nitrogen. Computational analysis has revealed important molecular descriptors:
Table 2: Molecular Descriptors of this compound[“]
Descriptor | Value |
LogP (octanol/water partition coefficient) | -1.02 |
LogD (octanol/buffer pH 6.0 partition coefficient) | -3.35 |
Polar Surface Area (PSA) | 130.61 A2 |
Non-Polar Surface Area (NPSA) | 168.25 A2 |
These measurements indicate that this compound exists as a bis-anion in a wide pH range, including physiological pH (6.0-7.4).
Solubility and Stability
This compound demonstrates remarkable solubility and stability properties:
Water Solubility: As a betalain pigment, this compound is highly water-soluble, which contributes to its bioavailability[“].
Amphiphilic Nature: With over 50% of its surface area being non-polar, this compound exhibits amphipathic characteristics. This property allows it to partition into phospholipid bilayers, particularly in dipalmitoyl-phosphatidyl choline (DPPC) with a binding constant of 3 × 10³ M⁻¹ at 37°C[“].
Membrane Interactions: this compound partitions at the interface between the hydrophilic head groups and hydrophobic core of DPPC bilayers, positioning it ideally for interactions with both aqueous peroxyl radicals and lipoperoxyl radicals[“].
LDL Binding: this compound can bind to low-density lipoproteins (LDL), with a maximum binding of 0.52 ± 0.08 nmol per mg LDL ApoB-100. This binding enhances LDL resistance to copper-induced oxidation[“].
Stability: this compound shows high digestive stability and bioaccessibility from cactus pear fruits. A study by Gómez-Maqueo et al. reported bioaccessibility values of 58 ± 5% for the Colorada (yellow-orangish) variety of Opuntia ficus-indica[“].
Case Study: Bioaccessibility in Human Consumption
A human dietary study demonstrated that after ingestion of cactus pear fruit pulp, 0.098 ± 0.012 nmol this compound per mg Apo B-100 (49 ± 8 millimol per mol Apo B-100) was found in LDLs isolated three hours post-consumption, corresponding to a peak plasma concentration of approximately 7 μM[“].
Spectroscopic Characteristics
This compound exhibits distinct spectroscopic properties that are crucial for its identification and quantification:
UV-Visible Absorption: this compound shows a characteristic absorption maximum at 482 nm, which is commonly used for its spectrophotometric analysis and quantification[“][“].
Fluorescence: While specific fluorescence data for this compound is limited in the provided search results, betalains generally exhibit fluorescence properties that can be used for their detection and analysis.
Electrochemical Properties: Voltammetric studies have revealed two characteristic peaks for this compound:
Peak at 611 mV
Peak at 895 mV
These peaks suggest reducing capacity against organic radicals commonly formed in cellular environments, including alkyl-peroxyl, lipoperoxyl, and hypervalent iron reactive species from heme-proteins1.
Research Findings: Antioxidant Activity
Spectrophotometric and electrochemical studies have demonstrated this compound's potent antioxidant properties:
ABTS Radical Scavenging: this compound was more effective than Trolox (a vitamin E derivative) at scavenging the ABTS cation radical[“][“].
Lipoperoxyl Radical Reduction: The pigment reduced lipoperoxyl radicals from linoleic acid methyl ester under oxidation by the 2,2′-azobis(2,4-dimethylvaleronitrile) (AMVN) azo-initiator, behaving as a classical chain-breaking antioxidant with effectiveness comparable to vitamin E.
Hypervalent-Iron Reduction: this compound effectively reduced the hypervalent-iron oxoferryl heme of perferryl-Hb, outperforming both Trolox and vitamin C in this capacity[“][“].
Biosynthesis of Indicaxanthin in Plants
Precursors and Pathways
The biosynthesis of indicaxanthin follows the betalain biosynthetic pathway, which originates from the amino acid tyrosine. The pathway can be divided into two main stages:
Formation of betalamic acid
Condensation of betalamic acid with amino acids or amines
Formation of Betalamic Acid
The first step in this compound biosynthesis is the formation of betalamic acid, which serves as the chromophore for all betalains, including this compound. This process involves the following steps:
Tyrosine is hydroxylated to form L-DOPA (L-3,4-dihydroxyphenylalanine)
L-DOPA undergoes extradiol cleavage to produce 4,5-seco-DOPA
4,5-seco-DOPA spontaneously cyclizes to form betalamic acid
Condensation Reaction
This compound is formed through the spontaneous condensation of betalamic acid with proline. This reaction occurs in the vacuole of plant cells.
Table 1: Key Steps in this compound Biosynthesis
Step | Substrate | Product | Enzyme |
1 | Tyrosine | L-DOPA | Tyrosinase or Polyphenol oxidase |
2 | L-DOPA | 4,5-seco-DOPA | DOPA 4,5-dioxygenase (DOD) |
3 | 4.5-seco-DOPA | Betalamic acid | Spontaneous |
4 | Betalamic acid + Proline | This compound | Spontaneous |
Enzymes Involved
Several key enzymes are involved in the biosynthesis of this compound:
Tyrosinase or Polyphenol oxidase: Catalyzes the hydroxylation of tyrosine to L-DOPA
DOPA 4,5-dioxygenase (DOD): Catalyzes the extradiol cleavage of L-DOPA to form 4,5-seco-DOPA
The DOD enzyme is particularly crucial in betalain biosynthesis, as it represents the committed step in the pathway. Studies have shown that DOD genes have been identified and characterized in various betalain-producing plants, including Beta vulgaris and Opuntia ficus-indica[“].
Regulation of Biosynthesis
The regulation of this compound biosynthesis is complex and involves various factors:
Transcriptional Regulation: The expression of genes encoding biosynthetic enzymes is regulated by transcription factors. For example, in Beta vulgaris, the R2R3-MYB transcription factor BvMYB1 has been shown to regulate betalain biosynthesis genes[“].
Environmental Factors: Various environmental stimuli can influence this compound biosynthesis. A case study on red prickly pears demonstrated that wounding and UVB light synergistically induce the postharvest biosynthesis of this compound and betanin. This suggests that stress conditions can upregulate the biosynthetic pathway.
Developmental Regulation: The production of this compound is often tissue-specific and developmentally regulated. For instance, in cactus pear fruits, the accumulation of this compound increases during fruit ripening.
Feedback Inhibition: While specific data on this compound is limited, studies on related pathways suggest that end-product feedback inhibition may play a role in regulating biosynthesis. For example, in the salicylic acid biosynthesis pathway, which shares the chorismate precursor with the betalain pathway, feedback inhibition by the end product regulates biosynthesis[“].
Research Findings:
A study on Opuntia ficus-indica revealed that the biosynthesis of betalains, including this compound, is influenced by various factors:
Light exposure significantly increased betalain content in cactus pear fruits.
Temperature stress (both high and low) induced betalain accumulation.
Mechanical wounding of the fruit peel led to increased betalain biosynthesis, likely as a defense mechanism.
These findings suggest that the regulation of this compound biosynthesis is closely tied to plant stress responses and environmental adaptation mechanisms.
Natural Sources
Cactus Pear (Opuntia ficus-indica)
Cactus pear, particularly Opuntia ficus-indica, is the most significant and well-studied source of indicaxanthin. This fruit contains high concentrations of the pigment, especially in its yellow-orange varieties.
Concentration and Distribution
The concentration of this compound in cactus pear varies depending on the cultivar and fruit color. Yellow-orange varieties typically contain higher levels of this compound compared to red varieties.
Table 1: this compound Content in Cactus Pear Varieties
Bioavailability and Stability
A study by Gómez-Maqueo et al. reported high bioaccessibility of this compound from Opuntia ficus-indica fruit extracts, with values of 58 ± 5% for the Colorada (yellow-orangish) variety. This high bioaccessibility contributes to the potential health benefits of consuming cactus pear fruits.
Human Absorption Study
A human dietary study demonstrated that after ingestion of cactus pear fruit pulp, this compound was detected in plasma, reaching a peak concentration of approximately 7 μM after three hours[“]. This finding highlights the bioavailability of this compound from cactus pear in humans.
Beets (Beta vulgaris)
While beets are primarily known for their betacyanin content, they also contain this compound, albeit in lower concentrations compared to cactus pear.
Concentration in Beets
The concentration of this compound in beets is generally lower than that of betacyanins. In fresh red beetroots, this compound (referred to as vulgaxanthin I in some sources) is present as one of the minor betaxanthins[“].
Table 2: Betalain Content in Red Beetroot
Variety | This compound Content |
Yellow-orange (Colorada) | High |
Red | Lower |
Varietal Differences
Different beetroot varieties show varying levels of betaxanthins, including this compound. A study comparing various Beta vulgaris varieties found that:
Beetroot varieties 'Monorubra' and 'Libero' had higher betacyanin content (9.69 and 8.42 mg/100 mL, respectively)
Other beet varieties contained lower levels of betacyanins: Sugar beet 'Labonita' (0.11 mg/100 mL), Swiss chard 'Lucullus' (0.09 mg/100 mL), fodder beet 'Monro' (0.15 mg/100 mL)9
While these figures represent total betacyanin content, they indicate the potential for varietal differences in this compound concentrations as well.
Mirabilis jalapa Flowers
Mirabilis jalapa, also known as the four o'clock flower, is another source of this compound.
Concentration and Extraction
A study on Mirabilis jalapa flowers revealed that the alkaloid fraction contained significantly higher levels of betaxanthins, including this compound, compared to the ethanol extract:
The alkaloid fraction had 6.17 times higher betaxanthin levels than the ethanol extract, as measured by UV-vis spectrophotometry
HPLC analysis showed 4 times higher betaxanthin levels in the alkaloid fraction compared to the ethanol extract[“].
Dominant Compound
LC-MS/MS analysis of the alkaloid fraction from Mirabilis jalapa flowers identified this compound as the most dominant compound[“]. This finding highlights the potential of Mirabilis jalapa as a concentrated source of this compound.
Other Plant Sources
While cactus pear, beets, and Mirabilis jalapa are the most studied sources of this compound, other plants within the Caryophyllales order may also contain this pigment.
Potential Sources
Amaranthus species: Some Amaranthus species have been reported to contain betaxanthins, which may include this compound[“]
Portulaca grandiflora: This plant, also known as moss rose, has been reported to contain betalains, potentially including this compound[“]
Rivina humilis: Berries of this plant have been found to synthesize betalains, including this compound[“]
Fungi Sources
Interestingly, some fungi of the genera Amanita, Hygrocybe, and Hygrophorus also contain betalains, which may include this compound. However, their role in fungi is unknown, and these are not considered practical sources for human consumption[“].
Extraction and Purification Methods
Indicaxanthin, a yellow betaxanthin pigment primarily found in cactus pear (Opuntia ficus-indica), has gained significant attention due to its unique properties and potential health benefits. This article explores the various extraction and purification methods used to obtain high-quality this compound, focusing on traditional extraction techniques, modern purification processes, and analytical methods for quantification.
Traditional Extraction Techniques
Traditional extraction methods for this compound typically involve solvent extraction techniques. These methods are widely used due to their simplicity and effectiveness.
Solvent Extraction
Solvent extraction is a common method used to isolate this compound from plant material. The process generally involves the following steps:
Plant material preparation: Cactus pear fruits, particularly the yellow cultivar, are selected and processed.
Solvent selection: Methanol or ethanol-water mixtures are commonly used.
Extraction process: The plant material is mixed with the solvent and subjected to agitation or maceration.
A typical extraction protocol involves:
Using a 1:1 ethanol-water mixture (v/v) acidified to pH 5.0 with 1% formic acid (v/v)
Mixing 2 grams of sample with 50 mL of extraction solvent
Maintaining the mixture in an orbital shaking incubator for 30 minutes at 30°C
Maceration
Maceration is a traditional extraction technique that involves soaking the plant material in a solvent for an extended period. While simple, it may not be as efficient as more modern techniques for extracting this compound.
Modern Purification Processes
Modern purification processes have significantly improved the yield and purity of extracted this compound.
Ultrasound-Assisted Extraction (UAE)
UAE has emerged as an efficient technique for extracting bioactive compounds from plant materials. This method enhances extraction yield and reduces processing time compared to conventional extraction methods.
A typical UAE protocol for this compound extraction involves:
Continuing extraction in an ultrasonic bath at 165 W and a frequency of 25 kHz for 30 minutes at 30°C
Centrifuging the mixture for 15 minutes at 30,000× g and 4°C
Collecting and combining the supernatants
Size Exclusion Chromatography
Size exclusion chromatography, particularly using Sephadex G-25, has been employed to separate this compound from other compounds in the initial extract. A typical process involves:
Packing a 30 × 3-cm chromatographic column with 35 g of Sephadex G-25
Dissolving the extract in a mixture of CH3OH/15% CH3OH with 0.05% CH3COOH (50:50, v/v)
Eluting with a flow rate of 3 ml/min, using 1% CH3COOH as the mobile phase
Solid-Phase Extraction (SPE)
SPE using C18 columns has been reported as an effective purification step for this compound. This technique helps remove impurities and concentrate the target compound.
High-Performance Liquid Chromatography (HPLC)
Semi-preparative HPLC is a powerful tool for obtaining highly pure this compound fractions. A typical method involves:
Using a C18 column
Employing a gradient elution system
Monitoring separations at 536 nm, 470 nm, and 280 nm to confirm the absence of other compounds
Analytical Methods for Quantification
Accurate quantification of this compound is crucial for assessing the efficiency of extraction and purification processes.
Spectrophotometric Analysis
Spectrophotometric analysis at 482 nm is commonly used for quantifying this compound. This method relies on the compound's characteristic absorption spectrum.
HPLC Analysis
HPLC coupled with diode array detection (DAD) is a precise method for analyzing this compound. It allows for both quantification and purity assessment of the compound.
A typical HPLC-DAD method for this compound analysis involves:
Using a Varian Microsorb C-18 column (4.6 × 250 mm)
Eluting with a 20-min linear gradient from solvent A (1% acetic acid in water) to 20% solvent B (1% acetic acid in acetonitrile)
Applying a flow rate of 1.5 mL/min
Detecting at 482 nm
Under these conditions, this compound typically elutes after 8.15 minutes.
Liquid Chromatography-Mass Spectrometry (LC-MS)
LC-MS, particularly ESI(+)-MS, provides accurate purity assessments of this compound preparations. This technique is highly sensitive and can detect trace amounts of impurities or degradation products.
Case Study: Extraction and Purification of this compound from Opuntia ficus-indica
A study by Gómez-Maqueo et al. demonstrated the effectiveness of combining traditional and modern extraction techniques for obtaining high-quality this compound from Opuntia ficus-indica var. Colorada (yellow-orange variety).
Table 1: Extraction and Purification Efficiency of this compound
Process Step | Yield |
Initial Extraction (30% ethanol + UAE) | 92.8% of total betaxanthins |
Semi-preparative RP-HPLC Purification | 1.74 ± 0.01 mg betanin/g dry weight |
Overall Yield | 41% of total betacyanin content |
This combined approach resulted in a higher yield and purity of this compound compared to previously reported methods, highlighting the benefits of integrating traditional and modern techniques.
Bioavailability and Metabolism
Intestinal Absorption Mechanism
Studies using Caco-2 cell monolayers have provided insights into the absorption mechanism of indicaxanthin:
Apparent permeability coefficient (Papp) in the absorptive direction: (4.4 ± 0.4) × 10⁻⁶ cm s⁻¹[“]
Transport is non-polarized, linear as a function of time and concentration
Unaffected by inhibitors of membrane transporters
Permeation increases remarkably after EDTA treatment of the cell monolayer, suggesting paracellular transport[“]
Distribution in Tissues
This compound's amphiphilic nature allows it to distribute across various tissues, including crossing the blood-brain barrier (BBB).
LDL Binding
This compound demonstrates a high affinity for low-density lipoproteins (LDL):
Maximum binding: 0.52 ± 0.08 nmol per mg LDL ApoB-100
In vivo binding: 0.098 ± 0.012 nmol per mg Apo B-100 (49 ± 8 millimol per mol Apo B-100) in LDLs isolated 3 hours post-consumption[“]
Brain Distribution
HPLC analysis of brain tissue 1 hour after oral administration of 2 μmol/kg this compound in rats revealed accumulation in:
Cortex
Hippocampus
Diencephalon
Brainstem
Cerebellum
Notably, this compound was not detected in the striato-pallidal complex[“].
Metabolic Pathways and Excretion
This compound undergoes minimal metabolic transformation in the body, with a significant portion excreted unchanged in urine.
Metabolic Stability
Studies have shown that this compound does not undergo significant metabolic transformation during intestinal absorption or systemic circulation[“]. This stability contributes to its high bioavailability and potential biological effects.
Excretion
The primary route of excretion for this compound is through urine:
76% of the ingested dose is excreted in urine within 12 hours[“]
The high urinary excretion rate suggests efficient renal clearance of the compound
Comparison with Other Betalains
This compound shows superior bioavailability compared to other betalains like betanin:
Table 2: Comparative Bioavailability of Betalains
Compound | Relative Bioavailability |
This compound | High |
Betanin | ~20-fold lower than this compound |
This difference is attributed to this compound's higher resistance to digestive degradation, greater absorption efficiency, and slower elimination rate[“]
Antioxidant Properties of Indicaxanthin
Free Radical Scavenging Activity
Indicaxanthin demonstrates remarkable free radical scavenging activity across various experimental models:
ABTS Radical Scavenging: this compound effectively scavenges the ABTS cation radical, outperforming Trolox (a vitamin E derivative) in spectrophotometric studies[“].
Lipoperoxyl Radical Reduction: The pigment reduces lipoperoxyl radicals from linoleic acid methyl ester under oxidation by the 2,2′-azobis(2,4-dimethylvaleronitrile) (AMVN) azo-initiator, behaving as a classical chain-breaking antioxidant.
Hypervalent-Iron Reduction: this compound effectively reduces the hypervalent-iron oxoferryl heme of perferryl-Hb, outperforming both Trolox and vitamin C in this capacity[“].
Comparison with Other Antioxidants
This compound's antioxidant capacity has been compared to several well-known antioxidants:
Table 1: Comparative Antioxidant Activity of this compound
Antioxidant | Relative Activity |
This compound | High |
Trolox | Lower than this compound in ABTS scavenging |
Vitamin C | Lower than this compound in hypervalent-iron reduction |
Vitamin E | Comparable in lipoperoxyi radical reduction |
In a study comparing various betalains, this compound showed superior antioxidant activity compared to betanin, particularly in protecting against lipid oxidation.
Mechanisms of Antioxidant Action
This compound's antioxidant mechanisms are multifaceted:
Electron Donation: Voltammetric studies reveal two characteristic peaks at 611 mV and 895 mV, suggesting reducing capacity against various organic radicals commonly formed in cellular environments[“].
Chain-Breaking Activity: this compound acts as a chain-breaking antioxidant in lipid peroxidation processes, interrupting the propagation of free radical reactions[“].
Metal Chelation: While not explicitly stated in the search results, many antioxidants exhibit metal chelation properties, which could be a potential mechanism for this compound.
Cellular Redox Modulation: this compound has been shown to modulate redox-dependent cellular pathways, contributing to its overall antioxidant effects[“].
In Vitro and In Vivo Studies
Several studies have demonstrated this compound's antioxidant properties in both in vitro and in vivo models:
In Vitro Studies:
Endothelial Cell Protection: this compound inhibited endothelial dysfunction in an in vitro model, showcasing its potential in protecting against oxidative stress-induced vascular damage[“].
Neuronal Protection: In olfactory ensheating nerve cells exposed to amyloid beta, this compound reduced O2•− and ROS production, demonstrating neuroprotective potential[“].
Cellular Redox Homeostasis: In various cell types, including Caco-2 and THP-1 cells, this compound restored cellular redox homeostasis under oxidative stress conditions[“].
In Vivo Studies:
Brain Antioxidant Effects: HPLC analysis of rat brain tissue 1 hour after oral administration of 2 μmol/kg this compound showed accumulation in various brain regions, suggesting potential neuroprotective effects[“].
Metabolic Disorder Protection: In a high-fat diet mouse model, this compound treatment reduced oxidative stress markers in liver and adipose tissue, demonstrating its potential in combating obesity-related oxidative stress[“].
Table 2: this compound's Antioxidant Effects in Various Models
Model | Observed Effects |
Endothelial cells | Inhibition of oxidative stress-induced dysfunction |
Neuronal cells | Reduction of ROS production |
Rat brain tissue | Accumulation in various brain regions |
High-fat diet mice | Reduction of oxidative stress markers in liver and adipose tissue |
Anti-inflammatory Effects
Studies have shown indicaxanthin's ability to modulate inflammatory pathways, suggesting potential applications in functional foods targeting inflammation-related conditions[“].
Neuroprotective Potential: Research indicates that this compound can cross the blood-brain barrier and accumulate in various brain regions, suggesting possible applications in functional foods for cognitive health[“].
Metabolic Health: A study on high-fat diet mice demonstrated this compound's potential in ameliorating glucose dysmetabolism and lipid disorders, indicating possible applications in functional foods for metabolic health[“].
Case Study: Metabolic Health Application
A study by Allegra et al. (2021) found that this compound treatment in high-fat diet mice:
Reduced body weight gain
Improved glucose tolerance
Decreased lipid accumulation in liver and adipose tissue
Reduced oxidative stress markers
These findings suggest potential applications in functional foods or nutraceuticals targeting metabolic disorders[“].
Challenges in Commercial Production
Despite its promising applications, the commercial production of this compound faces several challenges:
Source Limitation: this compound is primarily found in cactus pear fruits, which may limit large-scale production[“].
Extraction and Purification: Efficient extraction and purification methods need to be developed for industrial-scale production.
Stability Issues: Like other betalains, this compound is sensitive to various environmental factors, necessitating careful handling and storage.
Regulatory Approval: As a relatively new food colorant, this compound would require regulatory approval for widespread use in food products.
Cost-effectiveness: The production process needs to be optimized to make this compound competitive with existing natural and synthetic colorants.
To address these challenges, researchers are exploring various strategies:
Improved extraction techniques from cactus pear fruits
Development of synthetic or semi-synthetic production methods
Advanced encapsulation technologies to enhance stability
Exploration of alternative sources of this compound
Neuroprotective Properties
Blood-Brain Barrier Penetration
One of the most significant properties of indicaxanthin is its ability to cross the blood-brain barrier (BBB), a crucial factor for any compound intended to exert neuroprotective effects.
Kinetics and Distribution
A study by Allegra et al. (2015) demonstrated that this compound can cross the BBB and accumulate in the rat brain within 1 hour of oral administration at a dose of 2 μmol/kg, which is compatible with dietary consumption of cactus pear fruits in humans[“]. The study revealed:
Peak concentration: 20 ± 2.4 ng of this compound per whole brain after 2.5 hours
Elimination: First-order kinetics within 4 hours
Brain Distribution Pattern
HPLC analysis of rat brain tissue 1 hour after oral administration of 2 μmol/kg this compound showed accumulation in various brain regions[“]:
Table 1: Brain Distribution of this compound
Brain Region | Accumulation |
Cortex | Present |
Hippocampus | Present |
Diencephalon | Present |
Brainstem | Present |
Cerebellum | Present |
Striato-pallidal complex | Not detected |
This distribution pattern suggests that this compound may exert its neuroprotective effects in specific brain areas associated with cognitive functions and neurodegenerative disorders.
Effects on Neurodegenerative Processes
This compound demonstrates several mechanisms by which it may protect against neurodegenerative processes:
Antioxidant Activity
This compound exhibits potent antioxidant properties in the brain:
Reduces total reactive oxygen species (ROS) and superoxide (O2•−) production in neuronal cells[“]
Modulates redox-dependent cellular pathways[“]
Cancer Research
Antiproliferative Effects
Indicaxanthin has demonstrated significant antiproliferative activity against various cancer cell lines:
Colorectal Cancer:
In Caco-2 cells, this compound showed an IC50 of 115 ± 15 μM[“].
Antiproliferative effects were also observed in LOVO1, HCT116, and DLD1 cell lines[“].
Melanoma:
This compound exhibited antiproliferative effects in A375 human melanoma cells[“].
Cervical Cancer:
In HeLa cells, this compound showed an IC50 of approximately 150 μM[“].
Table 1: Antiproliferative Effects of this compound on Cancer Cell Lines
Cancer Type | Cell Line | IC50 |
Colorectal | Caco-2 | 115 ± 15 μM |
Cervical | HeLa | ~150 μM |
Importantly, this compound showed selectivity towards cancer cells, with no toxicity observed in post-confluent differentiated cells or normal human epidermal melanocytes at concentrations up to 200-250 μM[“].
Modulation of Cell Signaling Pathways
This compound modulates several key signaling pathways in cancer cells:
Epigenetic Modulation:
Induces demethylation of the p16INK4a gene promoter, reactivating its expression in Caco-2 cells[“].
Affects DNA methyltransferase (DNMT) expression and activity in colorectal cancer cells[“].
NF-κB Pathway:
Inhibits NF-κB activation in A375 melanoma cells[“].
Molecular modeling studies suggest that this compound can inhibit the active form of IKKβ, a key regulator of NF-κB signaling[“].
Cell Cycle Regulation:
Induces cell cycle arrest in the G2/M phase in Caco-2 cells[“].
Apoptosis Induction:
Increases expression of pro-apoptotic proteins (Bax, cytochrome c) and decreases anti-apoptotic proteins (Bcl-2) in combination with cisplatin in HeLa cells[“].
Autophagy Induction:
Upregulates autophagic markers LC3-II and Beclin1 in Caco-2 cells[“][“].
Potential as an Adjunct in Cancer Therapy
This compound shows promise as an adjunct in cancer therapy, particularly in combination with conventional chemotherapeutics:
Synergy with Cisplatin:
In HeLa cells, the combination of this compound and cisplatin demonstrated synergistic cytotoxic effects[“].
Pre-treatment with nutritionally relevant concentrations of this compound (2-10 μM) potentiated cisplatin activity up to threefold[“].
Chemosensitization:
The synergistic effect with cisplatin is mediated through oxidative stress-dependent mitochondrial cell death pathwayss[“].
Reduction of Side Effects:
The combination therapy approach allows for lower doses of conventional chemotherapeutics, potentially reducing toxicitys[“].
Current Research and Future Directions
Current research on this compound in cancer is focused on several areas:
Mechanism Elucidation:
Further studies are needed to fully understand the molecular mechanisms underlying this compound's anticancer effects, particularly its interaction with epigenetic regulators and cell signaling pathways.
In Vivo Studies:
While some animal studies have been conducted (e.g., xenograft model of cutaneous melanoma[“]), more in vivo research is required to validate the efficacy and safety of this compound.
Combination Therapies:
Exploring synergistic effects with other chemotherapeutics and targeted therapies is a promising avenue for future research.
Bioavailability and Pharmacokinetics:
Further studies on the bioavailability and pharmacokinetics of this compound in humans are needed to optimize its potential therapeutic use.
Clinical Trials:
Moving from preclinical to clinical studies will be crucial to establish the efficacy of this compound in cancer prevention and treatment.
工业应用
Use as a Natural Food Colorant
Indicaxanthin shows promise as a natural yellow food colorant, offering an alternative to synthetic dyes. Its potential applications include:
Beverages: this compound can be used to color beverages with neutral pH, such as flavored milk drinks[“].
Dairy Products: The pigment may be suitable for coloring dairy products like yogurt and ice cream.
Baked Goods: this compound could potentially be used in baking applications to provide a natural yellow color.
However, the use of this compound as a food colorant faces some limitations:
pH Sensitivity: It is not suitable for low pH beverages due to precipitation issues[“].
Color Stability: Like other betalains, this compound may be sensitive to light and heat.
To address these challenges, researchers have explored encapsulation techniques:
A study by Gandia-Herrero et al. demonstrated a successful encapsulation method for this compound:
Spray-drying with 20% maltodextrin at 140°C inlet temperature
Resulted in a bright yellow powder containing a single pigment
Improved stability, especially in the absence of light
Table 1: Stability of Encapsulated this compound
Condition | Stability |
pH 6-7 | High |
pH 4-5,8 | Lower |
Light exposure | Decreased |
Dark storage at 20°C | Stable for months |
This encapsulation technique significantly improved the stability of this compound, potentially expanding its applications in the food industry[“].
Potential in Nutraceuticals and Functional Foods
This compound's bioactive properties make it a promising candidate for nutraceuticals and functional foods:
Antioxidant Properties: this compound demonstrates potent antioxidant activity, often surpassing other well-known antioxidants like Trolox and vitamin C[“].
属性
IUPAC Name |
1-[2-(2,6-dicarboxy-2,3-dihydro-1H-pyridin-4-ylidene)ethylidene]pyrrolidin-1-ium-2-carboxylate | |
---|---|---|
Source | PubChem | |
URL | https://pubchem.ncbi.nlm.nih.gov | |
Description | Data deposited in or computed by PubChem | |
InChI |
InChI=1S/C14H16N2O6/c17-12(18)9-6-8(7-10(15-9)13(19)20)3-5-16-4-1-2-11(16)14(21)22/h3,5-6,10-11H,1-2,4,7H2,(H3,17,18,19,20,21,22) | |
Source | PubChem | |
URL | https://pubchem.ncbi.nlm.nih.gov | |
Description | Data deposited in or computed by PubChem | |
InChI Key |
RJIIQBYZGJSODH-UHFFFAOYSA-N | |
Source | PubChem | |
URL | https://pubchem.ncbi.nlm.nih.gov | |
Description | Data deposited in or computed by PubChem | |
Canonical SMILES |
C1CC([N+](=CC=C2CC(NC(=C2)C(=O)O)C(=O)O)C1)C(=O)[O-] | |
Source | PubChem | |
URL | https://pubchem.ncbi.nlm.nih.gov | |
Description | Data deposited in or computed by PubChem | |
Molecular Formula |
C14H16N2O6 | |
Source | PubChem | |
URL | https://pubchem.ncbi.nlm.nih.gov | |
Description | Data deposited in or computed by PubChem | |
Molecular Weight |
308.29 g/mol | |
Source | PubChem | |
URL | https://pubchem.ncbi.nlm.nih.gov | |
Description | Data deposited in or computed by PubChem | |
Physical Description |
Solid | |
Record name | Indicaxanthin | |
Source | Human Metabolome Database (HMDB) | |
URL | http://www.hmdb.ca/metabolites/HMDB0029386 | |
Description | The Human Metabolome Database (HMDB) is a freely available electronic database containing detailed information about small molecule metabolites found in the human body. | |
Explanation | HMDB is offered to the public as a freely available resource. Use and re-distribution of the data, in whole or in part, for commercial purposes requires explicit permission of the authors and explicit acknowledgment of the source material (HMDB) and the original publication (see the HMDB citing page). We ask that users who download significant portions of the database cite the HMDB paper in any resulting publications. | |
CAS No. |
2181-75-1 | |
Record name | Indicaxanthin | |
Source | Human Metabolome Database (HMDB) | |
URL | http://www.hmdb.ca/metabolites/HMDB0029386 | |
Description | The Human Metabolome Database (HMDB) is a freely available electronic database containing detailed information about small molecule metabolites found in the human body. | |
Explanation | HMDB is offered to the public as a freely available resource. Use and re-distribution of the data, in whole or in part, for commercial purposes requires explicit permission of the authors and explicit acknowledgment of the source material (HMDB) and the original publication (see the HMDB citing page). We ask that users who download significant portions of the database cite the HMDB paper in any resulting publications. | |
Melting Point |
160 - 162 °C | |
Record name | Indicaxanthin | |
Source | Human Metabolome Database (HMDB) | |
URL | http://www.hmdb.ca/metabolites/HMDB0029386 | |
Description | The Human Metabolome Database (HMDB) is a freely available electronic database containing detailed information about small molecule metabolites found in the human body. | |
Explanation | HMDB is offered to the public as a freely available resource. Use and re-distribution of the data, in whole or in part, for commercial purposes requires explicit permission of the authors and explicit acknowledgment of the source material (HMDB) and the original publication (see the HMDB citing page). We ask that users who download significant portions of the database cite the HMDB paper in any resulting publications. | |
Retrosynthesis Analysis
AI-Powered Synthesis Planning: Our tool employs the Template_relevance Pistachio, Template_relevance Bkms_metabolic, Template_relevance Pistachio_ringbreaker, Template_relevance Reaxys, Template_relevance Reaxys_biocatalysis model, leveraging a vast database of chemical reactions to predict feasible synthetic routes.
One-Step Synthesis Focus: Specifically designed for one-step synthesis, it provides concise and direct routes for your target compounds, streamlining the synthesis process.
Accurate Predictions: Utilizing the extensive PISTACHIO, BKMS_METABOLIC, PISTACHIO_RINGBREAKER, REAXYS, REAXYS_BIOCATALYSIS database, our tool offers high-accuracy predictions, reflecting the latest in chemical research and data.
Strategy Settings
Precursor scoring | Relevance Heuristic |
---|---|
Min. plausibility | 0.01 |
Model | Template_relevance |
Template Set | Pistachio/Bkms_metabolic/Pistachio_ringbreaker/Reaxys/Reaxys_biocatalysis |
Top-N result to add to graph | 6 |
Feasible Synthetic Routes
Q1: How does indicaxanthin exert its anti-inflammatory effects?
A1: this compound exhibits anti-inflammatory activity through multiple mechanisms. Research indicates it inhibits the activation of NF-κB, a key transcription factor involved in the inflammatory cascade [, , , ]. It also reduces the release of pro-inflammatory mediators such as IL-6, IL-8, prostaglandin E2 (PGE2), and nitric oxide (NO) [, ]. Moreover, this compound has been shown to inhibit the activation of NADPH oxidase (NOX-1) and reduce the generation of reactive oxygen species (ROS), both of which contribute to inflammation [].
Q2: Does this compound cross the blood-brain barrier, and if so, what are the implications?
A2: Yes, research has demonstrated that this compound can cross the blood-brain barrier (BBB) in rats [, ]. This finding is significant as it suggests that this compound could potentially exert direct effects on the central nervous system, opening avenues for exploring its potential in addressing neurodegenerative diseases.
Q3: What evidence suggests that this compound might have anti-cancer properties?
A3: this compound has demonstrated anti-proliferative and pro-apoptotic effects in various cancer cell lines, including colorectal, hepatic, and cervical cancer cells [, , , ]. Studies suggest that these effects are mediated, at least in part, through the inhibition of NF-κB signaling, induction of apoptosis, and modulation of DNA methylation [, , , ].
Q4: How does this compound interact with amyloid-beta, a key protein implicated in Alzheimer's disease?
A4: Research using olfactory ensheathing cells (OECs) suggests that this compound may modulate the expression levels and activity of tissue transglutaminase (TG2), an enzyme involved in amyloid-beta (Aβ) aggregation [, ]. This compound pre-treatment was shown to reduce the overexpression of TG2 induced by exposure to Aβ, potentially impacting Aβ aggregation and its downstream effects [, ].
Q5: What is the role of this compound in protecting red blood cells from oxidative damage?
A5: Studies have shown that this compound can be incorporated into red blood cells (RBCs), both after dietary consumption of cactus pear fruit and through ex vivo spiking of blood [, ]. Once inside the RBCs, this compound enhances their resistance to oxidative hemolysis, likely by acting as a radical scavenger and protecting cellular components from oxidative damage [, , ].
Q6: What is the molecular formula and weight of this compound?
A6: The molecular formula of this compound is C14H17N3O8, and its molecular weight is 351.3 g/mol [, ].
Q7: What are the key spectroscopic characteristics of this compound?
A7: this compound exhibits characteristic absorption maxima (λmax) in the UV-Vis spectrum. In its anionic form, the λmax is around 424 nm, which shifts to approximately 372 nm upon acidification []. These spectral properties are valuable for identifying and quantifying this compound in various matrices.
Q8: How stable is this compound under different storage conditions?
A8: this compound, like many natural pigments, can be susceptible to degradation under certain conditions. Studies have shown that its stability is affected by factors such as temperature, pH, light exposure, and the presence of metal ions [, , ].
Q9: What strategies have been explored to enhance the stability of this compound?
A9: Research indicates that this compound stability can be enhanced by storing it at low temperatures, in the dark, and under a nitrogen atmosphere []. Additionally, the addition of antioxidants such as ascorbic acid (vitamin C) can protect this compound from degradation [, ]. Encapsulation techniques, such as using double emulsion systems, have also shown promise in improving stability during storage [].
Q10: What is known about the bioavailability of this compound in humans?
A10: Research indicates that this compound is highly bioavailable in humans after consuming cactus pear fruit []. Studies have reported that peak plasma concentrations are reached within a few hours after ingestion, followed by a decline with a half-life of approximately 2.36 hours [].
Q11: Is this compound metabolized in the body, and if so, what are the metabolites?
A11: While the specific metabolic pathways of this compound in humans are not fully elucidated, research suggests that it undergoes some degree of metabolism. Further research is needed to identify the metabolites and understand their potential biological activities.
Q12: What in vitro models have been used to study the biological activity of this compound?
A12: Various in vitro models, including cell lines derived from different tissues (e.g., colorectal, hepatic, endothelial, and neuronal cells), have been employed to investigate the effects of this compound. These studies have provided valuable insights into its anti-inflammatory, antioxidant, cytoprotective, and anti-proliferative properties [, , , , , , ].
Q13: Has the efficacy of this compound been investigated in animal models of disease?
A13: Yes, studies have explored the effects of this compound in animal models. For instance, in a rat model of pleurisy (inflammation of the lining of the lungs), this compound was found to reduce inflammatory parameters []. In a mouse model of high-fat diet-induced obesity and insulin resistance, this compound administration showed beneficial effects on glucose metabolism and reduced oxidative stress and inflammation in the liver and adipose tissue [].
Q14: Have there been studies looking into targeted delivery strategies for this compound?
A14: While research on targeted delivery of this compound is limited, encapsulation techniques, like the double emulsion systems mentioned earlier, show promise in enhancing its stability, bioavailability, and potentially enabling targeted delivery to specific tissues [].
体外研究产品的免责声明和信息
请注意,BenchChem 上展示的所有文章和产品信息仅供信息参考。 BenchChem 上可购买的产品专为体外研究设计,这些研究在生物体外进行。体外研究,源自拉丁语 "in glass",涉及在受控实验室环境中使用细胞或组织进行的实验。重要的是要注意,这些产品没有被归类为药物或药品,他们没有得到 FDA 的批准,用于预防、治疗或治愈任何医疗状况、疾病或疾病。我们必须强调,将这些产品以任何形式引入人类或动物的身体都是法律严格禁止的。遵守这些指南对确保研究和实验的法律和道德标准的符合性至关重要。