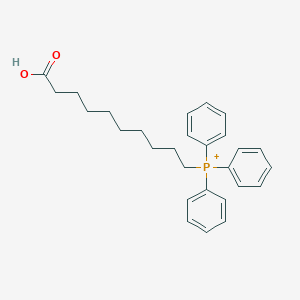
(9-Carboxynonyl)triphenylphosphonium
描述
AP219 是一种化学化合物,称为 (9-羧基壬基)三苯基鏻。它是一种内源性代谢物,也是 AP39 的对照化合物,AP39 是一种线粒体靶向硫化氢供体。 AP219 含有三苯基鏻支架,但缺少释放硫化氢的部分 .
准备方法
合成路线和反应条件
AP219 是通过将线粒体靶向基序三苯基鏻与脂肪族连接体偶联而合成的。合成路线包括以下步骤:
三苯基鏻支架的形成: 这涉及三苯基膦与适当的烷基卤化物反应形成三苯基鏻盐。
与脂肪族连接体的偶联: 然后将三苯基鏻盐与羧酸衍生物偶联,形成最终产物 AP219.
工业生产方法
AP219 的工业生产遵循类似的合成路线,但规模更大。反应条件经过优化,以确保最终产物的产率高和纯度高。该过程包括:
三苯基鏻盐的批量合成: 这是通过在合适的溶剂中使三苯基膦与烷基卤化物反应来实现的。
化学反应分析
反应类型
AP219 会经历几种类型的化学反应,包括:
氧化: AP219 可以发生氧化反应,其中它失去电子并形成氧化产物。
还原: 它也可以发生还原反应,其中它获得电子并形成还原产物。
常用试剂和条件
氧化: 常见的氧化剂包括高锰酸钾和过氧化氢。
还原: 常见的还原剂包括硼氢化钠和氢化锂铝。
形成的主要产物
从这些反应中形成的主要产物取决于所使用的具体条件和试剂。例如:
氧化: 主要产物是 AP219 的氧化衍生物。
还原: 主要产物是 AP219 的还原衍生物。
取代: 主要产物是 AP219 的取代衍生物.
科学研究应用
AP219 有几种科学研究应用,包括:
化学: AP219 用作涉及线粒体靶向硫化氢供体的研究中的对照化合物。
生物学: 它用于研究硫化氢对细胞生物能量学和线粒体功能的影响。
医学: AP219 用于与氧化应激及其对线粒体 DNA 完整性的影响相关的研究。
工业: 它用于开发具有特定性能的新化合物和材料.
作用机制
AP219 通过靶向线粒体发挥作用。三苯基鏻支架使其能够在线粒体内积累,在那里它可以与各种分子靶标相互作用。 该化合物不像 AP39 那样释放硫化氢,但它作为对照来研究三苯基鏻支架本身的影响 .
相似化合物的比较
类似化合物
AP39: 含有三苯基鏻支架和硫化氢释放部分的线粒体靶向硫化氢供体。
ADT-OH: AP39 中使用的硫化氢供体部分,没有线粒体靶向三苯基鏻基团.
AP219 的独特性
AP219 的独特之处在于它含有三苯基鏻支架,但缺少硫化氢释放部分。 这使其成为研究三苯基鏻支架本身影响的理想对照化合物,不受硫化氢释放的影响 .
生物活性
(9-Carboxynonyl)triphenylphosphonium (CNP) is a phosphonium compound that has garnered attention for its potential biological activities, particularly in the context of mitochondrial function and therapeutic applications. This article provides a comprehensive overview of its biological activity, including mechanisms of action, relevant case studies, and research findings.
Chemical Structure and Properties
CNP is characterized by a triphenylphosphonium moiety linked to a nonyl carboxylic acid. This structure allows it to act as a lipophilic cation, facilitating its accumulation within mitochondria due to the negative membrane potential of these organelles.
CNP primarily functions as a mitochondrial-targeted compound, influencing various biological processes:
- Mitochondrial Protection : CNP has been shown to protect against oxidative stress in mitochondria by promoting the generation of hydrogen sulfide (H2S), which has antioxidant properties. This effect is particularly relevant in conditions such as ischemia-reperfusion injury, where oxidative damage is prevalent .
- Antimycobacterial Activity : Research indicates that CNP exhibits significant antimycobacterial activity against strains such as Mycobacterium fortuitum and M. smegmatis. The compound's structure suggests that the aliphatic side chain plays a crucial role in its ability to inhibit mycobacterial growth .
Antioxidant Properties
Studies have demonstrated that CNP can selectively generate H2S within mitochondria. This process involves the hydrolysis of CNP to release H2S, which subsequently reacts with mitochondrial thiols to form persulfides. These reactions are crucial for mitigating oxidative stress and protecting mitochondrial integrity .
Antimycobacterial Activity
In vitro assays revealed that CNP derivatives possess varying degrees of activity against mycobacterial strains. For instance, compounds with longer aliphatic chains exhibited enhanced inhibitory effects, highlighting the importance of structural features in determining biological efficacy .
Case Studies
- Mitochondrial Targeting and Ischemia-Reperfusion Injury : A study explored the protective effects of CNP in a model of ischemia-reperfusion injury. Mice treated with CNP showed reduced markers of oxidative damage compared to controls, suggesting its potential as a therapeutic agent in acute injury scenarios .
- Antimycobacterial Efficacy : In research focused on mycobacterial infections, CNP demonstrated superior activity compared to standard treatments like ethambutol and isoniazid. The minimal inhibitory concentration (MIC) values indicated that modifications to the aliphatic chain could enhance activity further, making CNP a candidate for developing new antimycobacterial therapies .
Data Tables
Compound | MIC (mg/L) | Activity Type |
---|---|---|
(9-Carboxynonyl)TPP | 8 | Antimycobacterial |
Ethambutol | 16 | Standard Treatment |
Isoniazid | 32 | Standard Treatment |
属性
IUPAC Name |
9-carboxynonyl(triphenyl)phosphanium | |
---|---|---|
Source | PubChem | |
URL | https://pubchem.ncbi.nlm.nih.gov | |
Description | Data deposited in or computed by PubChem | |
InChI |
InChI=1S/C28H33O2P/c29-28(30)23-15-4-2-1-3-5-16-24-31(25-17-9-6-10-18-25,26-19-11-7-12-20-26)27-21-13-8-14-22-27/h6-14,17-22H,1-5,15-16,23-24H2/p+1 | |
Source | PubChem | |
URL | https://pubchem.ncbi.nlm.nih.gov | |
Description | Data deposited in or computed by PubChem | |
InChI Key |
VOOZXZVXPXRBBF-UHFFFAOYSA-O | |
Source | PubChem | |
URL | https://pubchem.ncbi.nlm.nih.gov | |
Description | Data deposited in or computed by PubChem | |
Canonical SMILES |
C1=CC=C(C=C1)[P+](CCCCCCCCCC(=O)O)(C2=CC=CC=C2)C3=CC=CC=C3 | |
Source | PubChem | |
URL | https://pubchem.ncbi.nlm.nih.gov | |
Description | Data deposited in or computed by PubChem | |
Molecular Formula |
C28H34O2P+ | |
Source | PubChem | |
URL | https://pubchem.ncbi.nlm.nih.gov | |
Description | Data deposited in or computed by PubChem | |
Molecular Weight |
433.5 g/mol | |
Source | PubChem | |
URL | https://pubchem.ncbi.nlm.nih.gov | |
Description | Data deposited in or computed by PubChem | |
Retrosynthesis Analysis
AI-Powered Synthesis Planning: Our tool employs the Template_relevance Pistachio, Template_relevance Bkms_metabolic, Template_relevance Pistachio_ringbreaker, Template_relevance Reaxys, Template_relevance Reaxys_biocatalysis model, leveraging a vast database of chemical reactions to predict feasible synthetic routes.
One-Step Synthesis Focus: Specifically designed for one-step synthesis, it provides concise and direct routes for your target compounds, streamlining the synthesis process.
Accurate Predictions: Utilizing the extensive PISTACHIO, BKMS_METABOLIC, PISTACHIO_RINGBREAKER, REAXYS, REAXYS_BIOCATALYSIS database, our tool offers high-accuracy predictions, reflecting the latest in chemical research and data.
Strategy Settings
Precursor scoring | Relevance Heuristic |
---|---|
Min. plausibility | 0.01 |
Model | Template_relevance |
Template Set | Pistachio/Bkms_metabolic/Pistachio_ringbreaker/Reaxys/Reaxys_biocatalysis |
Top-N result to add to graph | 6 |
Feasible Synthetic Routes
体外研究产品的免责声明和信息
请注意,BenchChem 上展示的所有文章和产品信息仅供信息参考。 BenchChem 上可购买的产品专为体外研究设计,这些研究在生物体外进行。体外研究,源自拉丁语 "in glass",涉及在受控实验室环境中使用细胞或组织进行的实验。重要的是要注意,这些产品没有被归类为药物或药品,他们没有得到 FDA 的批准,用于预防、治疗或治愈任何医疗状况、疾病或疾病。我们必须强调,将这些产品以任何形式引入人类或动物的身体都是法律严格禁止的。遵守这些指南对确保研究和实验的法律和道德标准的符合性至关重要。