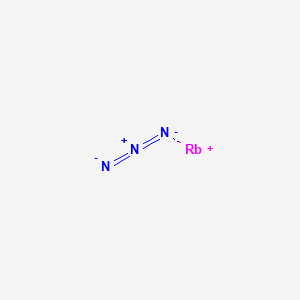
Rubidium azide (Rb(N3))
- 点击 快速询问 获取最新报价。
- 提供有竞争力价格的高质量产品,您可以更专注于研究。
描述
Rubidium azide, with the chemical formula RbN3, is an inorganic compound that serves as the rubidium salt of hydrazoic acid (HN3). It is characterized by its colorless, needle-like crystalline structure and is known for its explosive properties, similar to other azides. The compound has a molar mass of approximately 127.49 g/mol and a density of 2.79 g/cm³. Rubidium azide can be synthesized through various methods, including the reaction of rubidium sulfate with barium azide, which results in the formation of insoluble barium sulfate as a byproduct. Another method involves the reaction of butyl nitrite, hydrazine monohydrate, and rubidium hydroxide in the presence of ethanol. Due to its ability to decompose into rubidium metal and nitrogen gas when exposed to ultraviolet light, rubidium azide has potential applications in alkali vapor cells used in atomic clocks and magnetometers.
准备方法
Double Decomposition Reaction
Reactants Involved
One of the most common methods for synthesizing rubidium azide involves a double decomposition reaction between rubidium carbonate and sodium azide:
Rb2CO3+2NaN3→2RbN3+Na2CO3
This reaction results in the formation of rubidium azide while producing sodium carbonate as a byproduct.
Reaction Conditions
The reaction typically requires controlled conditions, including specific temperature and pressure settings, to ensure optimal yield. Generally, this reaction can be performed at room temperature, but careful monitoring is essential to achieve complete conversion.
Reactions with Rubidium Compounds
Reactants and Their Role
Another preparation method involves using rubidium sulfate combined with barium azide or lead(II) azide:
Rb2SO4+Ba N3)2→2RbN3+BaSO4
In this case, barium sulfate precipitates out of solution, allowing for easy separation from rubidium azide.
Product Isolation Techniques
After the reaction, isolation techniques such as filtration are employed to separate rubidium azide from the byproducts. The resulting product can then be purified through recrystallization from suitable solvents.
Alternative Methods of Synthesis
Hydrazine and Alkyl Nitrite Method
Rubidium azide can also be synthesized using hydrazine monohydrate and butyl nitrite in the presence of rubidium hydroxide:
C4H9ONO+N2H4·H2O+RbOH→RbN3+C4H9OH+3H2O
This method offers an alternative route that may yield high-purity rubidium azide under controlled conditions.
Benefits and Drawbacks of Each Method
Each preparation method has distinct benefits and drawbacks relating to factors such as yield, cost, and complexity. For example, while double decomposition reactions are straightforward, they may not always provide the highest purity levels compared to methods involving hydrazine.
Comparison of Yield and Purity
When comparing yields from various preparation methods, studies indicate that yields can vary significantly based on reactant quality and reaction conditions. Typically, double decomposition reactions yield moderate purity levels, while methods involving hydrazine can achieve higher purity but may require more stringent handling procedures.
Environmental and Economic Considerations
The environmental impact of synthesizing rubidium azide is an important consideration for researchers. Many preparation methods produce waste products that must be managed carefully to avoid environmental contamination. Economically, the cost of reagents and the complexity of synthesis routes can influence the overall feasibility of producing rubidium azide on a larger scale.
化学反应分析
Decomposition Reactions of Rubidium Azide
Thermal Decomposition
Rubidium azide undergoes thermal decomposition when heated, releasing nitrogen gas and forming rubidium metal. The reaction can be represented as:
2RbN3→2Rb+3N2
This reaction is significant because it demonstrates the explosive nature of rubidium azide when subjected to high temperatures, typically around 395 °C under normal conditions[“].
Shock-Induced Decomposition
In addition to thermal decomposition, rubidium azide is sensitive to mechanical shock. The impact sensitivity of rubidium azide is comparable to that of TNT, meaning that even minor disturbances can trigger its decomposition. This characteristic necessitates stringent safety measures during handling and storage[“].
Reactions with Other Compounds
Reaction with Water
When rubidium azide interacts with water, hydrolysis occurs, leading to the formation of rubidium hydroxide and nitrogen gas:
2RbN3+2H2O→2RbOH+3N2
This reaction highlights the compound's instability in aqueous environments and its potential for generating hazardous gases[“].
Interaction with Nitrogen Compounds
Rubidium azide can also react with other nitrogen-containing compounds, such as in the formation of rubidium nitride:
RbN3+N2→Rb3N
This reaction emphasizes the versatility of rubidium azide in forming various nitrogen-rich compounds under specific conditions[“].
Analytical Techniques for Studying Reactions
Spectroscopic Methods
Various analytical techniques are employed to study the reactions involving rubidium azide. Spectroscopic methods such as Nuclear Magnetic Resonance (NMR) and Infrared (IR) spectroscopy are crucial for analyzing reaction products and understanding reaction mechanisms. These techniques allow chemists to identify functional groups and molecular structures formed during reactions[“].
Chromatographic Techniques
Chromatography is another essential method used for separating and analyzing products from rubidium azide reactions. Techniques such as High-Performance Liquid Chromatography (HPLC) provide insights into the purity and composition of reaction mixtures, enabling detailed studies of reaction pathways[“].
Kinetics and Mechanisms of Reactions
Kinetic Studies
Kinetic studies are vital for understanding the rates at which reactions involving rubidium azide occur. Factors such as temperature, pressure, and concentration can significantly influence these rates. Experimental setups often include controlled environments to accurately measure reaction kinetics[“].
科学研究应用
Role in Atomic Physics and Quantum Mechanics
Use in Atomic Clocks
Rubidium azide plays a crucial role in the development of atomic clocks, which are essential for accurate timekeeping. Atomic clocks utilize the vibrations of rubidium atoms to measure time with extreme precision. The stability and frequency standards provided by rubidium azide are vital for global positioning systems (GPS) and telecommunications, where accurate timing is critical[“].
Applications in Quantum Computing
In the realm of quantum computing, rubidium azide is being investigated for its potential use in quantum bits (qubits). The unique properties of rubidium atoms allow for manipulation at quantum levels, making them suitable candidates for quantum information processing. Researchers are exploring how rubidium azide can enhance qubit performance and stability in quantum systems .
Applications in Laser Technology
Laser Cooling Techniques
Rubidium azide is utilized in laser cooling techniques, which are essential for achieving ultra-cold temperatures necessary for various experiments in physics. By using lasers to slow down atoms, researchers can study quantum phenomena at temperatures close to absolute zero. This technique is crucial for advancements in both fundamental physics and applied research .
Development of Laser Systems
The compound also contributes to the development of advanced laser systems. Rubidium azide can be used as a medium in certain types of lasers, enhancing their efficiency and output quality. These lasers find applications in fields ranging from medical technologies to telecommunications, showcasing the versatility of rubidium azide in laser technology[“].
Use in Alkali Vapor Cells
Composition and Functionality
Alkali vapor cells are critical components in various optical devices and sensors. Rubidium azide is integrated into these cells due to its favorable properties that facilitate the generation of alkali vapor when heated. This vapor plays a key role in applications such as magnetometry and atomic spectroscopy .
Advantages Over Other Materials
When compared to other alkali metals used in vapor cells, rubidium azide offers several advantages, including higher vapor pressure and better performance under specific experimental conditions. These benefits make it a preferred choice for researchers looking to optimize their experimental setups[“].
Contributions to Chemical Research
Reagent in Organic Synthesis
In organic chemistry, rubidium azide serves as a valuable reagent for synthesizing various compounds. Its ability to participate in nucleophilic substitution reactions allows chemists to create complex molecules efficiently[“].
Role in Click Chemistry
Rubidium azide is also employed in click chemistry, a powerful tool for bioconjugation and material science applications. This method allows for the rapid and selective coupling of molecules, facilitating advancements in drug development and materials engineering .
作用机制
Mechanism of Decomposition
Thermal Decomposition
One of the primary mechanisms by which rubidium azide acts is through thermal decomposition. When heated, rubidium azide decomposes into rubidium metal and nitrogen gas:
2RbN3→2Rb+3N2
This reaction occurs at elevated temperatures, typically around 395 °C, and releases nitrogen gas, which can be hazardous if not properly managed. The thermal stability of rubidium azide is a critical factor in its handling and application in various scientific contexts[“].
Shock-Induced Decomposition
In addition to thermal decomposition, rubidium azide is sensitive to mechanical shock. The impact sensitivity means that even minor disturbances can trigger its decomposition, similar to other high-energy azides. This characteristic poses risks during storage and handling but also highlights the compound's potential for use in specific applications where controlled decomposition is desired.
Interaction with Light
Ultraviolet Light Exposure
Rubidium azide can also undergo decomposition when exposed to ultraviolet (UV) light. The mechanism involves the absorption of UV radiation, which provides enough energy to break the bonds within the azide ion, leading to the release of nitrogen gas and other products. This photochemical behavior can be harnessed in experimental setups where controlled reactions are necessary[“].
Photochemical Reactions
Beyond simple decomposition, rubidium azide may participate in various photochemical reactions when exposed to light. These reactions can lead to the formation of different nitrogen-containing compounds or facilitate processes within alkali vapor cells used in atomic clocks and other precision instruments.
Role in Alkali Vapor Cells
Functionality in Vapor Cells
Alkali vapor cells are essential components in many optical devices and sensors, including atomic clocks. Rubidium azide plays a crucial role in these cells by providing a source of rubidium vapor when heated. This vapor is necessary for creating the conditions required for precise measurements in atomic physics.
Advantages Over Other Alkali Sources
Compared to other alkali metals used in vapor cells, rubidium azide offers several advantages, including higher vapor pressure and better performance at lower temperatures. These benefits enhance the efficiency and accuracy of devices that rely on alkali vapor cells, making rubidium azide a preferred choice for researchers[“].
相似化合物的比较
Overview of Similar Alkali Metal Azides
Lithium azide is an inorganic compound with the formula LiN3 and is characterized by its moderate solubility in water. It has a melting point of approximately 300 °C. Lithium azide is used in various applications, including as a reagent in organic synthesis and in the production of other azides.
Sodium azide is one of the most widely studied alkali metal azides, known for its high solubility in water (approximately 100 g/100 mL). It has a melting point of around 300 °C. Sodium azide is primarily used as a preservative in laboratories and as a propellant in automotive airbag systems due to its rapid decomposition into nitrogen gas upon heating.
Potassium azide has the chemical formula KN3 and features a melting point of about 340 °C. It is highly soluble in water and is often used in research settings for its explosive properties and as a reagent in organic synthesis. Its stability and reactivity make it valuable for various chemical reactions.
Cesium azide, represented by the formula CsN3, decomposes upon heating rather than melting at a specific temperature. It is soluble in water and exhibits moderate sensitivity to shock. Cesium azide's unique properties make it suitable for specific applications in research involving high-energy materials.
常见问题
What are the main differences between rubidium azide and sodium azide?
Rubidium azide has a higher melting point and lower solubility compared to sodium azide, which is highly soluble and widely used as a preservative.
How does the stability of rubidium azide compare to potassium azide?
Rubidium azide has a lower melting point than potassium azide but exhibits similar thermal sensitivity.
What are the implications of solubility differences among alkali metal azides?
Solubility affects their reactivity in solution-based reactions; higher solubility generally allows for more versatile applications.
Why is understanding the sensitivity of these compounds important?
Sensitivity impacts safety during handling and storage, influencing their suitability for various applications. This article provides a comprehensive overview comparing rubidium azide with similar alkali metal compounds, highlighting their unique properties, sensitivities, stabilities, and applications within scientific research contexts.
What is the primary mechanism by which rubidium azide decomposes?
The primary mechanism involves thermal decomposition into rubidium metal and nitrogen gas when heated.
How does mechanical shock affect rubidium azide?
Mechanical shock can trigger rapid decomposition, releasing nitrogen gas and posing safety risks.
What role does ultraviolet light play in the behavior of rubidium azide?
Ultraviolet light exposure can induce photochemical reactions leading to decomposition and formation of nitrogen gas.
Why is rubidium azide preferred in alkali vapor cells?
Rubidium azide provides higher vapor pressure and better performance compared to other alkali sources used in vapor cells. This article provides a comprehensive overview of the mechanism of action of rubidium azide, detailing its decomposition processes, interactions with light, and significance in scientific research applications while addressing common questions related to its behavior and uses.
属性
CAS 编号 |
22756-36-1 |
---|---|
分子式 |
N3R |
分子量 |
127.488 g/mol |
IUPAC 名称 |
rubidium(1+);azide |
InChI |
InChI=1S/N3.Rb/c1-3-2;/q-1;+1 |
InChI 键 |
GEWQYWRSUXOTOL-UHFFFAOYSA-N |
SMILES |
[N-]=[N+]=[N-].[Rb+] |
规范 SMILES |
[N-]=[N+]=[N-].[Rb+] |
22756-36-1 | |
产品来源 |
United States |
体外研究产品的免责声明和信息
请注意,BenchChem 上展示的所有文章和产品信息仅供信息参考。 BenchChem 上可购买的产品专为体外研究设计,这些研究在生物体外进行。体外研究,源自拉丁语 "in glass",涉及在受控实验室环境中使用细胞或组织进行的实验。重要的是要注意,这些产品没有被归类为药物或药品,他们没有得到 FDA 的批准,用于预防、治疗或治愈任何医疗状况、疾病或疾病。我们必须强调,将这些产品以任何形式引入人类或动物的身体都是法律严格禁止的。遵守这些指南对确保研究和实验的法律和道德标准的符合性至关重要。